Registration Dossier
Registration Dossier
Data platform availability banner - registered substances factsheets
Please be aware that this old REACH registration data factsheet is no longer maintained; it remains frozen as of 19th May 2023.
The new ECHA CHEM database has been released by ECHA, and it now contains all REACH registration data. There are more details on the transition of ECHA's published data to ECHA CHEM here.
Diss Factsheets
Use of this information is subject to copyright laws and may require the permission of the owner of the information, as described in the ECHA Legal Notice.
EC number: 232-140-5 | CAS number: 7789-00-6
- Life Cycle description
- Uses advised against
- Endpoint summary
- Appearance / physical state / colour
- Melting point / freezing point
- Boiling point
- Density
- Particle size distribution (Granulometry)
- Vapour pressure
- Partition coefficient
- Water solubility
- Solubility in organic solvents / fat solubility
- Surface tension
- Flash point
- Auto flammability
- Flammability
- Explosiveness
- Oxidising properties
- Oxidation reduction potential
- Stability in organic solvents and identity of relevant degradation products
- Storage stability and reactivity towards container material
- Stability: thermal, sunlight, metals
- pH
- Dissociation constant
- Viscosity
- Additional physico-chemical information
- Additional physico-chemical properties of nanomaterials
- Nanomaterial agglomeration / aggregation
- Nanomaterial crystalline phase
- Nanomaterial crystallite and grain size
- Nanomaterial aspect ratio / shape
- Nanomaterial specific surface area
- Nanomaterial Zeta potential
- Nanomaterial surface chemistry
- Nanomaterial dustiness
- Nanomaterial porosity
- Nanomaterial pour density
- Nanomaterial photocatalytic activity
- Nanomaterial radical formation potential
- Nanomaterial catalytic activity
- Endpoint summary
- Stability
- Biodegradation
- Bioaccumulation
- Transport and distribution
- Environmental data
- Additional information on environmental fate and behaviour
- Ecotoxicological Summary
- Aquatic toxicity
- Endpoint summary
- Short-term toxicity to fish
- Long-term toxicity to fish
- Short-term toxicity to aquatic invertebrates
- Long-term toxicity to aquatic invertebrates
- Toxicity to aquatic algae and cyanobacteria
- Toxicity to aquatic plants other than algae
- Toxicity to microorganisms
- Endocrine disrupter testing in aquatic vertebrates – in vivo
- Toxicity to other aquatic organisms
- Sediment toxicity
- Terrestrial toxicity
- Biological effects monitoring
- Biotransformation and kinetics
- Additional ecotoxological information
- Toxicological Summary
- Toxicokinetics, metabolism and distribution
- Acute Toxicity
- Irritation / corrosion
- Sensitisation
- Repeated dose toxicity
- Genetic toxicity
- Carcinogenicity
- Toxicity to reproduction
- Specific investigations
- Exposure related observations in humans
- Toxic effects on livestock and pets
- Additional toxicological data
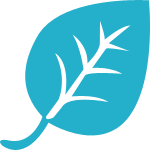
Endpoint summary
Administrative data
Description of key information
Additional information
Chromium (VI) has been shown to be taken up by a wide range of organisms from water, sediment and soil. For fish, although uptake does occur, the bioconcentration factors for chromium (VI) are usually very low (~1 l/kg). The following data and discussion are taken from the EU RAR (2005).
Fish
The uptake and accumulation of chromium by fish appears to be lower than for other aquatic organisms. Bioconcentration factors (BCFs) of around 1 l/kg have been determined for chromium (VI) using rainbow trout over 22-30 days exposure, with a value of 2.8 l/kg being reported in trout muscle for a longer exposure of 180 days (USEPA, 1980; Fromm and Stokes, 1962; Calamari et al., 1982). Camusso et al. (1995) carried out a study on the accumulation of chromium in fish. Caged rainbow trout (each 110-170 g weight) were exposed for 30 days at two sites on the river Po, one upstream and one downstream of a polluted tributary. Fish were removed periodically (at days 7,15 and 30) and analysed for the concentration of total chromium in gills, spleen, kidney, muscle and vertebral bone. Chromium was found to accumulate mainly in the spleen, muscles and gills, with the maximum increase in concentration being around 4 times that found in the pre-exposed fish in muscle. On an estimated whole body (wet weight) burden basis the total amount of chromium in the control fish was 18.7 mg, compared to the total amount of 21 and 32.9 mg in fish placed upstream and downstream of the tributary respectively.
Janus and Krajnc (1990) reported BCF values between 18 and 90 for rainbow trout that were exposed for 2 years in a lake polluted with chromates from cooling towers (as quoted in Braunschweiler et al., 1996). The effect of pH on the uptake and distribution of chromium (VI) in rainbow trout has been investigated by Van der Putte et al. (1981) as part of a series of three experiments to determine the mode of toxic action of chromium (VI). Fingerling fish were exposed to Na2 51CrO4 for 2-4 days at chromium concentrations between 2 and 50 mg Cr/l. All tissues reached equilibrium within the exposure period. Concentrations of 6.5 and 50.0 mg Cr/l were found to be lethal to the fish. Significantly more 51Cr was found in the fish exposed at a pH of 6.5 than at a pH of 7.8, but whole body BCFs were generally in the range 0.5-1 l/kg. The highest 51Cr concentrations were generally found in the gills at pH 6.5, but at pH 7.8, similar high concentrations as found in the gills were also found in the digestive tract, liver and kidney. When returned to clean water, 51Cr was rapidly lost from blood and digestive tract at pH 6.5, but less rapidly at pH 7.8. At pH 6.5, 85% of the chromium from whole body and 93% of the chromium from gills was eliminated in 3 days. In contrast, at pH 7.8, only 25% of the 51Cr was eliminated from whole body and 12% was eliminated from gills. The results indicated that pH was important in the uptake, tissue distribution and retention of chromium (VI) in rainbow trout (Van der Putte et al, 1981).
Buhler et al. (1977) examined the uptake and distribution of chromium in rainbow trout reared for two years in river water cotaining 0.25 µg Cr (VI)/l or between 1-10 µg Cr (VI)/l. Whole body residues of chromium of around 29 µg/kg wet weight and 18 µg/kg wet weight were determined in fish from the two exposures respectively, giving approximate whole body BCFs of the order of 18-116 l/kg. The highest concentrations of chromium were found in the opercular bone, spleen, liver, gastrointestinal tract and kidney. When the fish from the 1-10 µg Cr/l exposure group were exposed to a much higher concentration of chromium (VI) (2.5 mg Cr/l) for a short time period, a rapid increase in the whole body concentration was observed, with the concentrations in most tissues reaching equilibrium within 1 day. The whole body weight concentration reached 0.87 mg/kg wet weight after 22 days exposure, giving an estimated whole body BCF of around 0.4 l/kg. This additional chromium was not distributed as seen in the longterm exposures, but was concentrated in the cell cytosol, especially in the liver and kidney. The authors postulated that two different chromium ¿pools¿ might be present in fish, one giving concentrations of chromium in fish similar to levels found in water (giving BCFs ~1 l/kg), and one with a slower rate of exchange, leading to concentrations of total chromium in fish above the level in water. It was thought that these two ¿pools¿ could be related to rapid uptake of chromium (VI) with BCF~1 l/kg, followed by a slower reduction to chromium (III) in the fish. Similar two part uptake and elimination has been seen with marine polychaete worms (Hermione sp.) and freshwater clam (Lampallis radiata) (Towhill et al., 1978). The effect of fish bodyweight on the uptake and distribution of chromium (VI) (as potassium dichromate) has been studied in Goldfish (Carassius auratus) with bodyweights between 2 and 12 g. The tests were carried out at pH 7, at a temperature of 22oC and a chromium (VI) concentration of 20 mg Cr/l. The chromium solutions were renewed once per week over the 32-42 day exposure period. Some of the larger fish died at the exposure concentration used. Of the organs analysed, only the levels found in liver appeared to vary with exposure period, reaching a level of 773 mg/kg dry weight in large fish after 8 days and 1,315 mg/kg dry weight in small fish after 11 days. After this time period, the liver levels decreased slightly, but were still significantly elevated (¿=0.05) compared with controls. There was no significant difference in the liver levels for the small and large fish. The levels found in gills of exposed fish were significantly elevated compared with controls, and the levels found in small fish were significantly higher than those found in large fish. In bile, only the levels found in small fish were significantly elevated compared with controls (and large fish). The total chromium levels found in muscle of exposed fish were significantly higher than those of the controls, but no significant difference was seen between the large and small fish. Overall, no correlation between chromium levels and body weight could be determined in the study (Flos et al, 1983).
Mears and Eisler (1977) carried out a survey of total chromium levels in livers of marine fish (bluefish (Pomatomus saltatrix); tautog (Tautoga onitis); and tilefish (Lopholatilus chamaeleonticeps) collected off the New Jersey coast. The total chromium concentrations found in the livers were found to decrease with fish body length in female bluefish and male tautog, but no significant correlations (at the ¿=0.05 level) were seen in the total chromium concentrations in liver of fish of different sizes in the male bluefish, female tautog or male and female tilefish samples. As this was a field study, it is likely that the majority of chromium measured was chromium (III).
Giesy Jr. and Wiener (1977) analysed fresh water fish for levels of total chromium. The fish were taken from a lake where the average total chromium concentration of the water column was 0.35 µg/l. The mean whole body concentrations found in the fish (on a dry weight basis) were 0.16 mg/kg in bluegill (Lepomis macrochirus), 0.09 mg/kg in blueback herring (Alosa aestivalis), 0.28 mg/kg in brook silverside (Labidesthes sicculus), 0.19 mg/kg in golden shiner (Notemigonus crysoleucas) and 0.15 mg/kg in chain pickerel (Esox niger). Based on the measured concentrations of total chromium found in the water and fish, whole body BCFs in the range 260-800 l/kg on a dry body weight basis can be estimated (the mean dry weight/wet weight ratio of the fish was given as 0.22, so the fresh weight BCF values were 57-176 l/kg). Analysis of the stomach contents of chain pickerel indicated that this species was feeding mainly on bluegill. The authors concluded that, based on the measured concentrations in bluegill and chain pickerel, no bioaccumulation of total chromium was occurring through this food chain. As this was a field study, it is likely that much of the chromium present in the organisms in this study was as chromium (III).
Invertebrates
USEPA (1980) reported bioconcentration factors (BCFs) of between 125 and 200 l/kg for chromium (VI) in salt water with oyster (Crassostrea virginica), polychaete worm (Neanthes arenaceodentata) and blue mussel (Mytilus edulis) for exposures of 84-150 days. BCFs for chromium (III) in blue mussel (M. edulis) and soft shell clam (Mya arenaria) were generally lower at between 86 and 155 l/kg. Similar BCFs of 109-126 l/kg were determined by Shuster Jr. and Pringle (1969) for chromium (III) (as chromic nitrate) in oyster (Crassostrea virginica) exposed to 0.05 and 0.10 mg Cr/l using a flow-through sea water system (salinity 31¿) over 20 weeks.
Wang et al. (1997) studied the uptake of chromium (VI) by marine mussels (Mytilus edulis). The routes considered were direct uptake from water, feeding on alga/phytoplankton and feeding on sediments. Mussel bioconcentration factors (BCF) were derived by measuring the rate of uptake and rate of depuration of 51Cr (as sodium chromate; total concentration 2-200 nmol/l = 0.1-10 µg Cr/l) from seawater (salinity 28¿). For uptake via the water phase, a BCF (on a dry weight mussel basis) of 9,100 l/kg was determined for chromium (VI). The corresponding BCF for chromium (III) was 2,800 l/kg. Assimilation efficiencies were also determined for mussels fed on phytoplankton or sediment containing chromium (VI). The assimilation efficiency for chromium (VI) was 1.1-10.4% from phytoplankton (the assimilation efficiency for chromium (III) was lower at around 0.2-1.1%). In the experiments with sediments, the added chromium (VI) was found to be rapidly reduced to chromium (III) and so the assimilation efficiency of chromium (VI) could not be determined. The efflux rate constant of chromium (VI) from the mussels was found to be around 0.01 d-1. The authors used a kinetic model to analyse the bioaccumulation in mussels in the field and concluded that the major route of uptake of chromium (VI) is likely to be via the dissolved phase or via ingested food. This contrasted with the situation with chromium (III) where uptake via sediments was predicted to be the major route of uptake
In an earlier study (Wang and Fisher, 1996), the effect of food quality on the assimilation efficiency of chromium in mussels (Mytilus edulis) fed on varies species of algae was investigated. Seven alga species (Alexandrium tamarense, Chlorella autotrophica, Nannochloris atomus, Phaeodactylum tricornutum, Prorocentrum micans, Tetraselmis maculata and Thalassiosira pseudonana) were spiked with 51Cr (oxidation state not stated) and fed to mussels (shell length 3 cm) for 30 minutes (>90% of the food particles were ingested over this period). The pH of the seawater used in the experiments was around 8. After this feeding time, the retention of the 51Cr in the mussels was studied for 4 days. In this experiment, the assimilation efficiency was defined as the proportion of ingested metal retained after completion of digestion and gut evacuation (after approximately 72 hours). The results of the experiment indicated that >98% of the ingested 51Cr was excreted in faeces by 24 hours, indicating the chromium had a low potential for uptake and accumulation by mussels from food. The chromium assimilation efficiencies were all low (0.2-1.3%) for the 7 algal food species tested.
The uptake of chromium by mussels (Mytilus edulis) has also been studied by Walsh and O¿Halloran (1997). Groups of 20 mussels were exposed to various forms of chromium (concentration 50 µg Cr/l) over 4 weeks (solutions renewed every 3 days) in salt water (34.5¿) containing <5 mg/l of suspended solids. The exposure period was followed by a 48 hour depuration period (to ensure that no particulate chromium was present in the digestive tract) before the organisms were analysed for total chromium concentrations. The chromium species used in the experiment included potassium dichromate (chromium (VI)); and three forms of chromium (III): particulate Cr (OH)3 and soluble chromium (III) complexes with protein (typical of complexes found in leather tannery effluent) and citrate. The authors found that significant uptake of chromium (VI) from solution occurred in the digestive gland, gill and kidney. A similar pattern of uptake of total chromium has been seen in mussels (Mytilus edulis) from an estuary receiving wastewater from a leather tannery. The tannery processed around 4,000 hides per week and discharged around 85 kg of chromium/day, after primary precipitation treatment, to an estuary. The chromium present in the plant effluent consisted entirely of chromium (III) species. Mussels from around the plant had elevated levels of total chromium (levels in the gills were around 400-1,000 mg/kg dry weight; compared with levels of up to 6 mg/kg dry weight in gills of mussels from a reference site), with the levels found generally decreasing in the various tissues in the following order: gills>kidney>digestive gland>mantle>adductor muscle. A depuration half-life of 16-18 weeks was estimated for total chromium in gills and digestive gland, with longer half-lives estimated for other tissues. The majority of the chromium found was associated with the particulate fractions (rather than the cytosolic) fractions of the cells of gills and digestive glands. The authors concluded that two uptake patterns were occurring: over the short-term, uptake by ingestion dominated, resulting in high concentrations in the digestive gland; over the long term, preferential uptake and accumulation in the gills and kidney occurs (Walsh and O¿Halloran, 1998).
Chassard-Bouchaud et al. (1989) carried out a detailed microanalytical study of the distribution and chemical form of chromium (III) in mussels (Mytilus edulis) after exposure to chromium chloride in seawater (36¿) for 2 weeks. The uptake of chromium (III) was thought to occur via the gills in this study, with the main storage occurring in muscle. The digestive gland was thought to play a very minor role in the accumulation. The target organelle was the lysosome, where the metal was associated in an insoluble form with phosphorus and sulphur. Excretion of chromium (III) from the organism was thought to occur mainly via the kidney.
Chipman (1966) investigated the uptake of chromium (VI) (as Na2 51CrO4) and chromium (III) (as 51CrCl3 or 51Cr-EDTA chelate) from natural sea water (pH 8.05) by the clam Tapes decussatus, a representative of a detritus and particle feeding organism. In the experiments with chromium (III), a large proportion (80%) of the 51CrCl3 was found in the particulate phase immediately after addition to the seawater. The clams were shown to remove the chromium (III)- containing particles from solution by filter-feeding, and the peak levels of 51Cr found in the clams occurred within the first day of exposure. The level of chromium (III) in the clams then decreased with time during the 9-day exposure period as the clams excreted the ingested chromium (III)-containing particles and the amount of chromium (III) present in the sea water decreased. There was some evidence that the chromium (III) present in solution was adsorbed onto the surfaces of the clam (e.g. shells, gills, mantle etc.), but there was no evidence of uptake into the body tissues of chromium (III) from solution. In the experiments with chromium (III)- EDTA complex (where 99.75% of the chromium added was in solution), slight uptake of the chromium (III) was seen in shells and soft tissues over the 13-day exposure period, the concentrations found did not reach those present in the water i.e. BCF<1. In the chromium (VI) experiments, rapid uptake into body tissue was seen, with only a small amount of the chromium (VI) being found in the shells. After 20 days exposure, the concentration in body tissue was around 28 times that found in the water, although it is clear from the results that a steady state had not been reached within this time period. The depuration of the chromium (VI) from the clams was studied over a 39-day period. The results indicated that the rate of loss of the 51Cr from the clams was essentially the same as that for radioactive decay and so the depuration rate could not be determined. As part of a 2-generation assay test on a marine worm (Polychaeta, Neanthes arenaceodentata) a dose dependent accumulation of chromium (VI) was found. Juvenile worms (~1 cm in length) were exposed to various concentrations of chromium (VI) (2.6-38.2 µg/l as potassium dichromate) in filtered natural seawater at 18-24oC, salinity 33-35¿ and pH 8.1 using a static renew procedure (renewal every 3 weeks). At the highest chromium (VI) exposure level, 38.2 µg/l, the whole body tissue concentration of total chromium for the first generation was 8,278 µg/kg wet weight (BCF = 217 l/kg) after 158 days exposure, and 6,030 µg/kg wet weight (BCF = 158 l/kg) after 157 days exposure for the second generation (Oshida and Word, 1982).
The uptake of chromium (VI) (as Na2 51CrO4) and chromium (III) (as 51CrCl3) by barnacles (Balanus sp.) collected from a polluted estuary has been studied in laboratory experiments. The barnacles were exposed in sea water both with and without suspended particles (water filtered through 0.45 µm membrane). In the experiments with suspended particles, the barnacles were added to the system after equilibrium between the chromium in the dissolved and particulate phase had been reached (after 24 hours for chromium (III) and after 7 days for chromium (VI)). In filtered seawater a BCF of 543 l/kg on a dry tissue weight basis was determined for soft tissues for chromium (VI) after 27 days exposure, based on the total chromium concentration in the organism. The half-life for depuration of total chromium from the organism was estimated at 70 days. In the experiments with chromium (VI) in seawater containing 0.05 g/l of suspended particles, around 2% of the total chromium added to the test solution was associated with the particulate phase, and a BCF of 380 l/kg on a dry weight basis was determined for soft tissues after 61 days, with a depuration half life for the adsorbed chromium of 180 days. In the experiments with chromium (III), maximum uptake was seen after 15-20 days, coinciding with the complete removal of the radioactive particles (the chromium (III) formed a precipitate in the seawater solution) from solution by the barnacles. No evidence for accumulation of chromium (III) in the soft tissues of the organisms was seen (the chromium (III) passed through the digestive system without absorption (van Weerelt et al., 1984). A comparison of the accumulation of chromium (VI) (as Na2 51CrO4) by direct uptake from water with uptake from food (51Cr adsorbed onto Chlamydomonas sp after five days incubation) has been carried out using the American oyster Crassostrea virginica over 110 hours exposure in artificial seawater. More 51Cr was found to be accumulated by direct adsorption from the water than by ingestion of the algae and the rates of uptake by the two routes were also found to differ. The elimination of the 51Cr from the organisms was slow, with no elimination being detected over 5 days. The Na2CrO4 concentration in direct adsorption experiments was 6.62.10-4 mg/l (Preston, 1971).
A model of biota sediment accumulation factors (BSAF) relating the ratio of metal concentrations in 2 marine bivalves (Crassostrea virginica and Mytilus edulis) to sediment metal concentrations indicates total chromium has the smallest BSAF at 0.01 (Thomann et al., 1995). Crayfish, Procambarus clarkii, were exposed over 96 hours at 19.5oC to chromium (VI) (as sodium chromate) at concentrations ranging from 10 to 500 mg Cr/l. The tissue concentrations of total chromium found after exposure are shown in Table 3.13. The amount of chromium accumulated increased with increasing exposure concentration in all tissues, with the concentration of total chromium in gland > gills > hepatopancreas > muscle. However, the relative accumulation rates decreased with increasing chromium (VI) concentrations in the water (Hernandez et al., 1986). From the data reported, BCFs of around 4.3-7.4 l/kg can be estimated for based on the dry whole body weight values.
Stackhouse and Benson (1989) studied the effects of humic acid on the bioaccumulation of chromium (VI) (as potassium dichromate) and two forms of chromium (III) (as chromic chloride and a Cr-lignosulphonate complex) by Daphnia magna. In the experiment, 7-day old Daphnia were exposed to a chromium concentration of 10 mg Cr/l for 96 hours (solutions renewed every 24 hours) and the animals were then analysed for the presence of total chromium. The water used in the study had a hardness of 92 mg/l as CaCO3 and a pH of 8, and contained varying concentrations of humic acids (0, 0.5, 5 and 50 mg/l). After 96 hours exposure, the total chromium concentration in the chromium (VI)-exposed organism was between 58.5 and 67.8 mg Cr/kg dry weight at humic acid concentrations between 0 and 5 mg/l, and 52.1 mg Cr/kg dry weight at a humic acid concentration of 50 mg/l. These concentrations allow bioconcentration factors of 4,810-6,380 l/kg dryweight to be estimated for chromium (VI) (the background level of total chromium in the Daphnia was 4 mg/kg dry weight). In the chromium (III) uptake experiments, the humic acid had a much more marked effect in reducing the amount of chromium accumulated in the Daphnia (in the experiments with CrCl3, the concentration in Daphnia was reduced from 105.9 mg Cr/kg dry weight when no humic acid was present (BCF~10,190 l/kg dry weight) to 32.8.
Algae and aquatic plants
The uptake of chromium (VI) from water by 4 species of phytoplankton (Chlorella autotropica, Prorocentrum minimum, Tetraselmis levis and Thalassiosira pseudonana) has been studied by Wang et al (1997). Cells of each species (initial concentration 2-8.104 cells/ml) were exposed to 51Cr (VI) (as sodium chlorate; concentration 0.3 nmol/l) in seawater. Steady state concentrations were obtained after 3-10 days exposure (when the cell growth had reached the stationary phase. The concentration factors (on a cell dry weight basis) were 500 l/kg for C. autropica, 420 l/kg for P. minimum, 190 l/kg for T. levis and 470 l/kg for T. pseudonana. The equivalent concentration factors for chromium (III) were around a factor of 100-1,000 higher (12,000-130,000 l/kg). Cellular fractionation showed that >98% of the chromium (III) present on the cells was adsorbed onto the cell wall/membrane.
Jouany et al. (1983) determined the uptake of chromium (VI) (as potassium dichromate) by the freshwater alga Chlorella vulgaris as part of a 96h-toxicity study. The exposure concentrations used were 100-900 µg Cr (VI)/l, and the concentration factors determined were in the range 612-988 l/kg (based on the total chromium content of algae on a dry cell weight basis). The uptake of both chromium (III) (as chromium potassium sulphate, Cr (III)-EDTA complex or Cr (III)-glycine complex) and chromium (VI) (as potassium dichromate) by the fresh water green alga Chlorella pyrenoidosa and a wild strain of Chlorella have been studied by Meisch and Schmitt-Beckmann (1979). In the experiments, algal cultures were exposed to chromium concentrations of 0.5 and 1 mg Cr/l in nutrient medium for 5 days. After the exposure period, the total chromium concentrations in the dry algal cells were determined. The concentration factors for C. pyrenoidosa (on a dry cell weight basis) were around 256-390 l/kg for chromium (VI) and 558-580, 11-12 and 224-254 l/kg for the three chromium (III) species. Slightly lower concentration factors (220 l/kg for chromium (VI)) were obtained in the wild Chlorella strain.
Aksu et al. (1990) investigated the uptake of chromium (VI) (as potassium dichromate) by dead cells of Chlorella vulgaris. The maximum rate of uptake of chromium (VI) by the cells was found to occur over the temperature range 35-50oC and a pH of 1-2, however, these results have limited applicability to the environment. Other bioconcentration factors of 2,300-29,000 l/kg have been reported for natural algal populations exposed to chromium (VI) concentrations of 0.01-0.4 mg/l for 2-4 weeks (Braunschweiler et al., 1996) and BCFs of 20-215 l/kg have been estimated for chromium in alga collected in the river Rhine (Janus and Krajnc, 1990; as quoted in Braunschweiler et al., 1996).
Kähkönen and Manninen (1998) studied the uptake of chromium (VI) (as sodium chromate) by the aquatic plant Elodea canadensis. The plants were exposed to chromium (VI) for 24 hours in filtered lake water (pH 7.2-9.1). The mean initial exposure concentrations used in the experiment were 150, 800 and 2,090 µg Cr/l and these concentrations were found to remain reasonably constant throughout the exposure period. The concentration of total chromium found in the plants at the end of the experiments was 5.5 mg/kg dry weight in the control plants, and 8.3, 35 and 84 mg/kg dry weight in plants exposed to the 150, 800 and 2,090 µg Cr/l solutions respectively. Based on these data, bioconcentration factors (on a dry plant weight basis) of approximately 19-38 l/kg can be estimated.
For the risk assessment, a reliable value for the BCF in fish is needed. The available data indicate that the bioconcentration factor for chromium (VI) in fish is relatively low at around 1 l/kg. Once in the organism, reduction of chromium (VI) to chromium (III) appears to occur, resulting in an accumulation of total chromium in the organisms to a factor of approximately 100 times the original concentration in water. Uptake of chromium (III) directly from water is likely to be very low due to the limited water solubility and strong adsorption to sediment under most conditions found in the environment. Thus for the risk assessment, the following BCFs will be used: a) to estimate the concentration of chromium (VI) in fish; [Cr(VI)]fish mg/kg = BCFC (VI) = 1 l/kg [Cr(VI)]water mg/l b) to estimate the concentration of chromium (III) in fish resulting from uptake and subsequent reduction of chromium (VI); [Cr(III)]fish mg/kg = BCFCr(VI)-Cr(III) = 100 l/kg [Cr(VI)]water mg/kg. The uptake of chromium by aquatic organisms may be pH dependent, but insufficient information is available to take any possible variation in the BCF into account. Any pH dependence may be related to differences in solubility and speciation of the chromium in solution and this is taken into account to some extent in the consideration of bioavailability (e.g. adsorption, solubility) of the various forms elsewhere in the assessment. For chromium (VI) in solution at pHs found in the environment the two main species are likely to exist are the monovalent hydrochromate anion (HCrO4 -) which will dominate at lower pHs and the dichromate anion (Cr2O7 2-), which will dominate at higher pHs and higher chromium concentrations. It has been postulated that, due to its higher negative charge, the mobility of the dichromate anion across biological membranes is impaired relative to that of the hydrochromate anion (Walsh and O Halloran, 1997; Nair and Krishnamurthi, 1991b).
The uptake of chromium by other organisms appears to be higher than seen for fish, although few if any of the experiments distinguish between chromium (VI) and chromium (III) concentrations in the organisms. Similar to the situation for fish, it is possible that once taken up by the organism, chromium (VI) is reduced to chromium (III) in the tissues, resulting in a build up of chromium (III) and hence an overestimate for the true bioconcentration factor for chromium (VI). BCFs of up to around 9,100 l/kg (on a mussel dry weight basis) for chromium (VI) and 2,800 l/kg (on a mussel dry weight basis) for chromium (III) have been determined in mussels, and BCFs of around 500 l/kg (on a cell dry weight basis) for chromium (VI) and 12,000-130,000 l/kg (on a cell dry weight basis) for chromium (III) have been determined in algae. Transfer of chromium via the alga ¿ bivalve, and sediment ¿ bivalve food chains appears to be relatively low.
Uptake from soil (porewater)
The equilibrium partitioning of total chromium between soil and earthworms has been investigated by Janssen et al. (1997b). In the experiments, earthworms (Eisenia andrei) were exposed to samples of 20 soils that where known to contain various heavy metals. Soil samples (~1 kg) were placed in covered glass jars and acclimated to the experimental temperature of 20oC for 24 hours. Groups of 10 animals (approximately 21-24 weeks old) were then placed in each exposure vessel. After 3 weeks exposure the animals were collected and analysed for the presence of total chromium (after the animals had voided their gut contents). Bioconcentration factors (BCFs) were determined as: total metal concentration in worm (mmol/kg dry weight)/total metal concentration in solid phase (mmol/kg dry weight). The uptake of total chromium by the earthworms was generally found to be small, with bioconcentration factors in the range 0.03-0.53 being determined. The authors investigated the effects of various soil parameters, and also the possible species present in the soil pore water, on the uptake of total chromium. No significant correlation was found for the uptake of chromium by earthworm with any of the soil (e.g. pH, organic matter content, iron and aluminium oxyhydroxide contents, clay content and soil pore water properties (metal speciation, dissolved organic carbon, ionic strength), although some correlation of BCF with soil organic matter content was found in soils with a pH>5. Overall, from the soils studied, it appears that the BCF for earthworm is relatively independent of the soil properties, and that the total concentration of chromium in earthworm is governed mainly by the total concentration present in the soil. The results were thought to be consistent with uptake being via the pore water phase.
Van Gestel et al. (1993), as part of an earthworm reproduction test, also reported low bioconcentration factors for chromium by earthworms. In this experiment chromium (III) nitrate was added to artificial soil and 9.5-15.5 week old earthworms were incubated in the soil for 3 weeks at 20oC. The bioconcentration factors determined (on a [mg/kg dry worm]/[mg/kg dry soil] basis) were 0.031-0.047 at exposure concentrations of 10-100 mg Cr/kg dry soil, and 0.016-0.019 at 320-1,000 mg Cr/kg dry soil. At the end of a three week recovery period, where the earthworms were placed in clean soil, the total chromium levels had returned to background levels. The elimination half-life for total chromium was estimated at 51-109 days for the lowest exposure groups and 5-7 days for the highest exposure groups.
Sharma (1997) studied the uptake and distribution of chromium in maize (Zea mays L, Cv. Ganga 5) grown in refined sand under glass house conditions. Thirty days after sowing, sodium dichromate was added to the plants in the daily nutrient solution at concentrations of 0.05-1 mM and the concentration of total chromium present in various parts of the plant were determined after 46 days growth. The chromium uptake was found to be concentration dependent and greatest in the roots.
The uptake of chromium (VI) (as chromium trioxide) and chromium (III) (as chromic chloride) by barley (Hordeum vulgare) and rape (Brassica napus) has been studied in nutrient culture as part of a toxicity study. The plants (2 week old for barley; 3 week old for rape) were grown in a mineral solution (solution pH=5.0) containing chromium (concentration 10, 30, 50 or 100 mg Cr/l) for between 1 and 14 days. After the exposure period, plants were harvested and the leaves and stems were analysed for the concentration of total chromium. Effects on growth of the plants, particularly the roots, were seen at all concentrations tested. In the plants exposed to chromium (III), the peak concentration of total chromium reached 400 mg/kg dry weight (leaves) and 200 mg/kg dry weight (stems) in barley and 100 mg/kg dry weight (leaves) and 400 mg/kg dry weight (stems) in rape after 4-6 days exposure to chromium (III) at 100 mg/l. Exposure of the plants to chromium (VI) in solution resulted in plant concentrations around 1-2 orders of magnitude higher than seen for chromium (III). The concentrations of total chromium found in leaves of both plants were in the range 3,000-5,000 mg/kg dry weight when exposed to a chromium (VI) concentration of 100 mg/l. At this high chromium (VI) concentration significant toxic effects (growth reduction) were seen. At lower chromium (VI) concentrations (e.g. 10 mg/l) the levels found in leaves were around 100 mg/kg dry weight (Hauschild, 1993).
Otabbong (1990) looked at the effects of Si(OH)4 on the uptake of chromium (VI) (as chromium trioxide) by ryegrass (Lolium perenne). Two soils were used in the experiment. One soil had a pH of 5.2 and a clay and humus content of 36.1% and 7.0% respectively, and the second had a pH of 6.9 and a clay and humus content of 54.3% and 3.4% respectively. The soil was spiked with chromium (VI) at a concentration of 50 mg/kg dry weight and then sowed with seeds. After 30 days growth, the roots and shoots were analysed for the presence of total chromium. Plant growth in the chromium (VI) exposed soil was slightly inhibited when compared with the control plants, but the addition of Si(OH)4 caused a severe reduction in growth. Using the data for the chromium experiments (without the added Si(OH)4, soil-plant concentration factors (defined as concentration of total chromium (corrected for control) in plant (mg/kg dry weight)/concentration of chromium (VI) added to soil (mg/kg dry weight) of 1.4.10-4 and 2.6.10-3 for shoots and 0.0122 and 3.9.10-3 for roots
Information on Registered Substances comes from registration dossiers which have been assigned a registration number. The assignment of a registration number does however not guarantee that the information in the dossier is correct or that the dossier is compliant with Regulation (EC) No 1907/2006 (the REACH Regulation). This information has not been reviewed or verified by the Agency or any other authority. The content is subject to change without prior notice.
Reproduction or further distribution of this information may be subject to copyright protection. Use of the information without obtaining the permission from the owner(s) of the respective information might violate the rights of the owner.
