Registration Dossier
Registration Dossier
Data platform availability banner - registered substances factsheets
Please be aware that this old REACH registration data factsheet is no longer maintained; it remains frozen as of 19th May 2023.
The new ECHA CHEM database has been released by ECHA, and it now contains all REACH registration data. There are more details on the transition of ECHA's published data to ECHA CHEM here.
Diss Factsheets
Use of this information is subject to copyright laws and may require the permission of the owner of the information, as described in the ECHA Legal Notice.
EC number: 500-209-1 | CAS number: 68412-54-4 1 - 2.5 moles ethoxylated
- Life Cycle description
- Uses advised against
- Endpoint summary
- Appearance / physical state / colour
- Melting point / freezing point
- Boiling point
- Density
- Particle size distribution (Granulometry)
- Vapour pressure
- Partition coefficient
- Water solubility
- Solubility in organic solvents / fat solubility
- Surface tension
- Flash point
- Auto flammability
- Flammability
- Explosiveness
- Oxidising properties
- Oxidation reduction potential
- Stability in organic solvents and identity of relevant degradation products
- Storage stability and reactivity towards container material
- Stability: thermal, sunlight, metals
- pH
- Dissociation constant
- Viscosity
- Additional physico-chemical information
- Additional physico-chemical properties of nanomaterials
- Nanomaterial agglomeration / aggregation
- Nanomaterial crystalline phase
- Nanomaterial crystallite and grain size
- Nanomaterial aspect ratio / shape
- Nanomaterial specific surface area
- Nanomaterial Zeta potential
- Nanomaterial surface chemistry
- Nanomaterial dustiness
- Nanomaterial porosity
- Nanomaterial pour density
- Nanomaterial photocatalytic activity
- Nanomaterial radical formation potential
- Nanomaterial catalytic activity
- Endpoint summary
- Stability
- Biodegradation
- Bioaccumulation
- Transport and distribution
- Environmental data
- Additional information on environmental fate and behaviour
- Ecotoxicological Summary
- Aquatic toxicity
- Endpoint summary
- Short-term toxicity to fish
- Long-term toxicity to fish
- Short-term toxicity to aquatic invertebrates
- Long-term toxicity to aquatic invertebrates
- Toxicity to aquatic algae and cyanobacteria
- Toxicity to aquatic plants other than algae
- Toxicity to microorganisms
- Endocrine disrupter testing in aquatic vertebrates – in vivo
- Toxicity to other aquatic organisms
- Sediment toxicity
- Terrestrial toxicity
- Biological effects monitoring
- Biotransformation and kinetics
- Additional ecotoxological information
- Toxicological Summary
- Toxicokinetics, metabolism and distribution
- Acute Toxicity
- Irritation / corrosion
- Sensitisation
- Repeated dose toxicity
- Genetic toxicity
- Carcinogenicity
- Toxicity to reproduction
- Specific investigations
- Exposure related observations in humans
- Toxic effects on livestock and pets
- Additional toxicological data
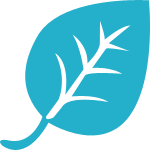
Biodegradation in water and sediment: simulation tests
Administrative data
Link to relevant study record(s)
Description of key information
Numerous studies have been conducted looking at degradation of NPEOs, mainly longer-chain ethoxylates, and NP in fresh or marine water and sediment. A comprehensive review of this data was published by Klecka et al. (2008). Overall, the data suggests that longer chain NPEOs degrade rapidly to shorter chain NPE-1 and NPE-2, as well as to the corresponding carboxylates. NP did not appear to be a significant breakdown produc in many of the sudies. The short chain NPEOs and the carboxylates break down slower, but complete mineralisation may at least in part occur. Microbial acclimation to the substances is required for optimal degradation efficiencies. Temperature is expected to play a role in degradation speed, with longer half-lives in colder conditions.
Testing with NP shows rapid degradation under oxic conditions and slower degradation in sediment with lower redox potential or under anaerobic conditions. In the well aerated sediment, NP isomers with short side chains and/or bulky alpha-substituents were found to be more recalcitrant to degradation. The degree of branching was found to correlate best with isomer half-life. Again, temperature is expected to play a role in the degradation kinetics. It is expected that the more water soluble (and ionized) 1 and 2 EO carboxylate derivatives will largely remain in the aqueous phase.
Key value for chemical safety assessment
Additional information
Freshwater and sediment
Aerobic biodegradation of NPEO was investigated in a laboratory-scale bioreactor (glass column filled with glass beads as carrier material and an aerated 10 L storage tank filled with water collected from the River Rhine. The bioreactor was spiked with two different technical mixtures of nonylphenol ethoxylates with an ethoxylate range of 2 - 9 and 4 – 15 at a concentration of 10 mg/L. Small amounts of octylphenol ethoxylates (OPEO) and decylphenol ethoxylated were present in the mixtures. The experimental duration was 31 days. Incubation temperature was not specified. After 4 days, 99% of the NPEO mixtures were dissipated (primary degradation). Nonylphenol carboxylates (NPEC) were identified as the main group of metabolites. The concentration of NPEC increased until Day 5 and subsequently decreased. Further degradation of NP1-2EC by a carboxylation of the alkylchain was observed (CAmPEnC with m = 5 - 9, n = 0 or 1). Both short-chain NPEC and CAPEC metabolites were still present in the bioreactor after 31 days. No change in initial NP was observed during the experiment. NP was not found as a metabolite in the study (Jonkers N et al., 2001).
Static die away tests were run with NP and NPE-1 in various river sediments. The effects of acclimation, mixing and temperature on the biodegradation rate were examined. For static systems incubated at 30°C, half-lives for four different sediments ranged from 13.6 – 99.0 days for NP and 69.3 – 115.5 days for NPE-1. Acclimation of the sediment microorganisms to NP or NPE-1 decreased the half-lives from 20.4 to 5.1 days and from 23.0 to 5.7 days, respectively. Temperature was a significant factor in controlling the degradation rate of both NP and NPE-1. Half-lives for NP were 40.8, 4.2 and 3.0 days and for NPE-1 were 57.8, 6.0 and 4.7 days in samples incubated at 20, 40 and 50°C, respectively. Mixing was also shown to be an important determinant. Shaking the flasks decreased the half-lives by a factor of about 2 – 5 as compared to static systems (Yuan SY et al., 2004). In the static systems, anaerobic conditions were likely established in the sediments, which have been shown to retard or limit the biodegradation of NP.
Aerobic biodegradation of 14C-NPE-9 was examined in the laboratory (5 L glass tanks) under condition simulating a river water environment. Water was collected from the Missouri river near Columbia, Ohio (USA), downstream from a wastewater treatment plant. After filtration, the water was amended with clarified secondary effluent from the wastewater treatment facility.14C-NPE-9 was added to three biodegradation test systems at a concentration of 100 µg/L. A fourth test system was similarly prepared, but was dosed with a mixture of non-labelled NP and NPEO oligomers with an ethoxylation grade of 1, 4 and 9 (at a concentration of 100 µg/L NP/NPEO) to simulate the fate of partially degraded NPE in sewage treatment outfall. The reactors were maintained at ca. 20°C. Changes in oligomer distribution and mineralization to14CO2 were monitored for 128 days. After 128 days, over 40% of 14C-NPEO aromatic ring was converted to 14CO2 and another 21% was incorporated in the biomass. Primary degradation of the NPEO (conversion to metabolites other than NP, NP ethoxylates and NP carboxylates) was estimated to be 87-97% at study end. NP was a minor metabolite, accounting for less than 0.4% of the initial NPEO. The study showed the opening, metabolisation and mineralisation of the phenolic ring of NPEO in the environment (Naylor CG et al., 2006).
The biodegradability of 19 different NP isomers (technical mixture of NP isomers with branched side chains) was investigated in two river sediments under oxic and anoxic conditions. The surface sediments (0 – 5 cm) were grab-sampled from the Santa Ana River in Southern California (USA), at one site in the upper river (Sediment A) and one site in the middle segment of the river (Sediment B). Both sediments were free of detachable NP residues at test start. The aerobic and anaerobic incubation trials were conducted in 125 ml amber vials with 10 g (dry weight equivalent) of sediment spiked with 50 µl of a 1,000 mg/L NP solution. All samples were incubated at ca. 21°C. Biodegradation of NP isomers in Sediment A under oxic conditions was generally efficient and isomer-specific. NP isomers underwent an initial phase (14 – 21 days) of fast degradation, with half-lives ranging from 0.9 to 13.2 days, followed by a slower second phase. Sediment B had a lower redox potential than sediment A, reflective of nitrate reducing conditions. This was likely due to higher organic carbon and lower sand content. In sediment B, NP isomers underwent stagnant degradation during the first 7 – 14 days, followed by faster degradation with half-lives varying from 15.1 to 20.1 days. The overall half-lives in sediment B were longer than those in sediment A. In the well aerated sediment, NP isomers with short side chains and/or bulky alpha-substituents were found to be more recalcitrant to degradation. The degree of branching was found to correlate best with isomer half-life (Lu Y and Gan J, 2013).
Estuarine/marine water and sediment
Biotransformation of NPE-10 (a commercial mixture containing ethoxylates in the range of 1-18 EO, with an average of 10 EO) by estuarine mixed bacterial cultures was analyzed under laboratory conditions using a static die-away method. The experiments were performed with autochtonous bacterial cultures from brackish and saline water layers from the highly stratified Krka River estuary of the Croatian Mid-Adriatic region. Experiments were conducted in 5 L glass vials incubated at temperatures corresponding to those found in the environment at sampling time (13 – 22.5°C). NPEO was added at test start at 0.1 and 1 mg/L. Biotransformation kinetics of mixed bacterial culture from the brackish water layer were faster than those from the saline water layer at all temperatures examined and at both concentrations of NPEO (0.1 and 1 mg/L). This was probably due to a better pre-adaptation of the brackish water bacteria to NPEOs in their natural habitat. Under winter temperature conditions (13°C), the estimated dissipation half-life (DisT50) ranged from 23 - 69 days, while the DisT50 under summer temperature conditions (22.5°C) ranged from 2.5 - 35 days. Transformation to NPEC was not followed and the main intermediate formed during the experiment was NPE-2 (Kveštak R and Ahel M, 1995).
A static dissolved organic carbon (DOC) die-away test was performed with estuarine water from four sampling sites in Tampa Bay, FL (USA). Water samples were collected at 30 cm below the surface, mid-water and 30 cm above the bottom. Depending on sampling site (middle of the Bay, port area and tidal river), the temperature ranged from 27.5 to 31.0°C. The water was then transferred to 10 L glass reagent bottles used for the DOC die away experiments. NPEO was added at a nominal concentration of 4 mg/L of a commercial formulation with an ethoxylation grade of 18 (NPE-18). Incubations were conducted in the dark at 28°C for 183 days. The concentration of NPE-2, NPE-1, the 1 and 2EC carboxylates, NP and total surfactant were monitored at intervals of 4-8 days for 89 days and at 30-day interval thereafter until 183 days. Complete primary degradation of NPE-18 was detected after 4 - 24 days with an adaption time between 0 and 12 days. The formation of NPE-2 reached its maximum concentration in 4 - 16 days. The 2EC carboxylate increased until Day 20 - 76 with little or no decrease until the end. Smaller amounts were detected for NPE-1 (<0.1 mg/L) and the 1EC carboxylate (maximum concentration 20% of NPE-2). NP was not measured (detection limit 0.01 mg/L). It was estimated that approximately 36 - 56% of the surfactants converted to CO2 and H2O or other metabolites. However, this was not confirmed analytically (Potter TL et al., 1999).
The aerobic and anaerobic biodegradation of 14C-labelled NPE-4 (14 µg/g dw) was examined in estuarine sediments collected from locations (in the USA) know to contain NPE and NP residues. Test vessels maintained under aerobic conditions were incubated for 116 days, whereas tests under anaerobic conditions were maintained for 129 days. Mineralisation to14CO2was monitored using LCMS, while concentrations of NPE oligomers were measured with HPLC and ESI-MS/MS. Based on changes in total concentrations of all14C-labelled NP and NPE, biodegradation half-lives measured under aerobic and anaerobic conditions were 85 and 287 days, respectively. The formation of 14C-NP was not detected in either test. Although such findings could be expected for biodegradation of NPE-4 under aerobic conditions, they are in contradictions to those reported elsewhere for anaerobic conditions. It is possible that insufficient time had passed for degradation to NP to occur. NPE carboxylates were formed under both conditions. Only small amounts of the parent compound were mineralised to14CO2 under aerobic conditions (<2% of applied radioactivity), possibly because of sorption to the highly organic rich sediment used or to inhibitory effects of other organic contaminants and heavy metals known to be in the sediment (Ferguson PL and Brownawell BJ, 2003).
A study was conducted to determine the biodegradation of NP in a seawater/sediment simulation test.14C-labelled NP, at a concentration of 11 µg/L, was incubated (2 L glass flasks) for 16 weeks in seawater alone or in seawater/sieved soft bottom sediment at 11± 2°C in darkness with shaking twice a week for one minute. Formalin was added to the four flasks containing seawater and half of the flasks containing seawater and sediment were bubbled with nitrogen gas prior to the start of the experiment. The seawater used was collected below the halocline in a less polluted coasta area in Sweden. Samples were taken after 1, 2, 4 and 8 weeks and, for control flasks without sediment, after 16 weeks. The formed labelled CO2 was collected and quantified with the help of a scintillation counter. In flasks containing formalin, no CO2 was recovered, indicating that any14CO2 came from the nonylphenol in the presence of microorganisms. In the absence of sediment, the initial degradation was very slow but when microorganisms had become adapted, after 4 weeks, the degradation rate increased rapidly. This suggested that a period of adaptation was required. After 8 weeks, approximately 50% of 14C from NP was found in the CO2 fraction. No further degradation was observed between 8 and 16 weeks. In the presence of sediment, the initial degradation rate was high and did not increase after longer incubation. After 8 weeks, approximately 40% of the14C from NP was found in the CO2 fraction. Lack of oxygen reduced the degradation rate by half in the presence of sediment (Ekelund R et al., 1993).
Degradation of pure grade NP was studied in marine sediment and seawater collected from the coastal area near Adelaine (South Australia). The seawater experiments were run in aereated 2.5 L bottles spiked with 5 µg/L of NP. Incubation temperature was ca. 20°C and test duration 56 days. Non-aerated conditions were also tested in 1 L bottles at 5 µg/L NP. The sediment experiments used 5 mg sediment in 5 ml of seawater and 1 µg/L NP. Incubation temperature was ca. 20°C and test duration 70 days. Aerobic testing occurred in 100 mL bottles, anaerobic testing in 16 x 125 mm culture tubes. NP degraded rapidly in seawater (< 1 week) due to biotic as well as abiotic factors with no lag phase. Under aerobic conditions, the half-life of NP in sediment was 5.8 days. Under anaerobic conditions, little or no degradation occurred in the sediment (Ying GG and Kookana RS, 2003).Information on Registered Substances comes from registration dossiers which have been assigned a registration number. The assignment of a registration number does however not guarantee that the information in the dossier is correct or that the dossier is compliant with Regulation (EC) No 1907/2006 (the REACH Regulation). This information has not been reviewed or verified by the Agency or any other authority. The content is subject to change without prior notice.
Reproduction or further distribution of this information may be subject to copyright protection. Use of the information without obtaining the permission from the owner(s) of the respective information might violate the rights of the owner.
