Registration Dossier
Registration Dossier
Data platform availability banner - registered substances factsheets
Please be aware that this old REACH registration data factsheet is no longer maintained; it remains frozen as of 19th May 2023.
The new ECHA CHEM database has been released by ECHA, and it now contains all REACH registration data. There are more details on the transition of ECHA's published data to ECHA CHEM here.
Diss Factsheets
Use of this information is subject to copyright laws and may require the permission of the owner of the information, as described in the ECHA Legal Notice.
EC number: 231-674-6 | CAS number: 7681-65-4
- Life Cycle description
- Uses advised against
- Endpoint summary
- Appearance / physical state / colour
- Melting point / freezing point
- Boiling point
- Density
- Particle size distribution (Granulometry)
- Vapour pressure
- Partition coefficient
- Water solubility
- Solubility in organic solvents / fat solubility
- Surface tension
- Flash point
- Auto flammability
- Flammability
- Explosiveness
- Oxidising properties
- Oxidation reduction potential
- Stability in organic solvents and identity of relevant degradation products
- Storage stability and reactivity towards container material
- Stability: thermal, sunlight, metals
- pH
- Dissociation constant
- Viscosity
- Additional physico-chemical information
- Additional physico-chemical properties of nanomaterials
- Nanomaterial agglomeration / aggregation
- Nanomaterial crystalline phase
- Nanomaterial crystallite and grain size
- Nanomaterial aspect ratio / shape
- Nanomaterial specific surface area
- Nanomaterial Zeta potential
- Nanomaterial surface chemistry
- Nanomaterial dustiness
- Nanomaterial porosity
- Nanomaterial pour density
- Nanomaterial photocatalytic activity
- Nanomaterial radical formation potential
- Nanomaterial catalytic activity
- Endpoint summary
- Stability
- Biodegradation
- Bioaccumulation
- Transport and distribution
- Environmental data
- Additional information on environmental fate and behaviour
- Ecotoxicological Summary
- Aquatic toxicity
- Endpoint summary
- Short-term toxicity to fish
- Long-term toxicity to fish
- Short-term toxicity to aquatic invertebrates
- Long-term toxicity to aquatic invertebrates
- Toxicity to aquatic algae and cyanobacteria
- Toxicity to aquatic plants other than algae
- Toxicity to microorganisms
- Endocrine disrupter testing in aquatic vertebrates – in vivo
- Toxicity to other aquatic organisms
- Sediment toxicity
- Terrestrial toxicity
- Biological effects monitoring
- Biotransformation and kinetics
- Additional ecotoxological information
- Toxicological Summary
- Toxicokinetics, metabolism and distribution
- Acute Toxicity
- Irritation / corrosion
- Sensitisation
- Repeated dose toxicity
- Genetic toxicity
- Carcinogenicity
- Toxicity to reproduction
- Specific investigations
- Exposure related observations in humans
- Toxic effects on livestock and pets
- Additional toxicological data
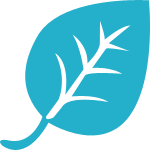
Hydrolysis
Administrative data
Link to relevant study record(s)
Description of key information
In contact with water the substance will hydrolyse rapidly and form a pre-equilibrium. This first step is a first-order reaction with a rate constant of 3.0 s-1 at 20 °C and a pH value of 5. In principle, this reaction can be summarized as I2 + H2O = HOI + I- + H+. As hydronium ions are formed in this reaction it can be expected that natural environmental conditions support the formation of hypoiodous acid (HOI) and iodide. In the second step of the hydrolysis HOI disproportionates and forms iodide and iodate via a mechanism not fully understood. This step is considered as a second-order reaction and is clearly slower taking at least tens of minutes depending on the prevalent conditions. Additionally, the findings in the different studies indicate that the initial reaction of the second disproportionation is relative fast, but then after about one hour the gradient of the rate constants flatten reaching for steady-state conditions in solutions at pHs between 6 and 8. Anyhow, it can be assumed that at that time the major partition of molecular iodine is already degraded. The overall hydrolysis can be summarized as 3 I2 + 3 H2O = IO3- + 5 I- + 6 H+.
Since the first disproportionation and the fast initial step of the second equilibrium reaction play a decisive role in the environmental fate of iodine and adverse effects of HOI in the environment cannot be excluded the rate constant of the first reaction is considered for the risk assessment.
Key value for chemical safety assessment
- Half-life for hydrolysis:
- 0.01 min
- at the temperature of:
- 12 °C
Additional information
The hydrolysis of iodine has been in the focus of the scientific community for several decades. From various studies it has become clear that it is a very complex system consisting of competing partial reactions forming two equilibria in principle and a vast number of different iodine species which are formed and consumed in different pathways.The overall hydrolysis can be summarized as 3 I2 + 3 H2O = IO3- + 5 I- + 6 H+. Hypoiodous Acid (HOI) seems to be a key intermediate in the whole system.
In 1955 Allen et al. examined the hydrolysis of iodine and determined the equilibria constant for the formation of hypoiodous acid for two different temperatures (K= 5.4 * 10-13, 25 °C and K= 0.49x10-13, 1.6 °C) by photometric determination of the iodine and triiodide concentration in the solution. Based on previous observations of Liebhafsky (Liebhafsky, 1931) and Morgan (Morgan, 1954) they postulated a reaction system for the formation of hypoiodous acid consisting of different competing partial reactions.
I2 + H2O = H2OI+ + I-
I2 + H2O = HOI + H+ + I-
I2 + I- = I3-
Eigen and Kustin in 1962 studied this reaction system by a temperature-jump relaxation technique. They extended the system of competing partial reactions for the formation of hypoiodous acid with additional potential routes and iodine species and were able to propose a general mechanism. Additionally, they were able to determine a rate constant for the first pre-equilibrium (formation of HOI) at a pH value of 5 (k= 3.0 s-1).
In 1980 Thomas and co-workers studied the hydrolysis of iodine and disproportionation of hypoiodous acid to iodide and iodate by a combination of photometrical and potentiometrical analytical techniques for pH values between 7 and 12. Their experimentally found rate constants were in good agreement to their numerical integration of the theoretical reaction kinetics. They determined the extent of the disproportionation by comparing the initial iodine concentration and the concentration of the formed iodine species. About 25 % of the injected iodine remained after 4 days at pH 7, 1% remained after 14 hours at pH 8 and about 0.1% after 2 hours at pH 9. For higher pH values the ratio of initial iodine and formed iodide concentrations approached the theoretical limit, but did not reached it.
Truesdale et al. examined the disproportionation of hypoiodous acid at pHs between 7 and 13 without addition of iodide. Based on their results they were able to develop a model of a second order kinetics depending on the hypoiodous acid concentration influenced by two competing pre-equilibria involving iodine and hypoiodous acid as well as hypoiodous acid and hypoiodite. Because the rate maximum appeared at ca. pH 9.5 he assumed a central role of hypoiodous acid in the disproportionation mechanism as this species pre-dominates the system at this pH value.
Such as Thomas et al. Truesdale observed the formation of a steady-state condition of the disproportionation for pHs between 6 and 8 after a reasonably fast initial reaction. The extent of the initial reaction decreases with decreasing pH. While at pH 6.82 only 21% of the iodine is converted the extent increases to 93% at pH 8.33 within the first hour (Truesdale, 1995). Afterwards a phase of a distinctly decreased reaction rate is established which is indicated by a brown color of the solution that can remain for days or even weeks at 25° C. Likewise, Luther and Sammet (Luther, 1905) reported a period of 49 days to reach equilibrium at pH 6.2. Sugawara and Terada (Sugawara, 1958) have observed that iodate was still being produced in sea water after 25 days.
In general the iodine - hypoiodous acid equilibrium is very fast as Eigen and Kustin showed, and takes a fraction of a second at a temperature of 20 °C and pH value of 5 to adjust. Thus, it can be expected that most of the iodine in the environment is rapidly transformed as the natural pH value actually promotes the pre-equilibrium reaction. In contrast, the step of the disproportionation of hypoiodous acid to iodide and iodate is much slower, taking at least tens of minutes to reach completion at 25 °C. Additionally, a strong connection with the pH value for this reaction is observable reaching higher reaction rates for readily alkaline environment. However, about 90% of all iodine found in natural freshwater occurs as iodide (FOREGS Geochemical Baseline Programme (FGBP) database).
References:
Liebhafsky HA (1931). Reactions involving hydrogen peroxide, iodine and iodate ion. IV. The Oxidation of iodine to iodate ion by Hydrogen Peroxide, J. Am. Chem. Soc., 53, 2074-2090.
Luther R (1905). Sammet GV, Z. Elektrochem. 11, 293.
Morgan KJ (1954). Some reactions of inorganic iodine compounds., Quart. Rev., 8, 123-146.
Truesdale VW, Canosa-Mas C (1995). Kinetics of Disproportionation of Hypoiodous Acid in Phosphate and Borate Buffer at pH<8.5 modelled using Iodide Feedback, J.Chem. Soc. Faraday Trans., 91(15), 2269 -2273.
Sugawara K, Terada K (1958). Oxidised iodine in sea water, Nature, 182, 250.
Information on Registered Substances comes from registration dossiers which have been assigned a registration number. The assignment of a registration number does however not guarantee that the information in the dossier is correct or that the dossier is compliant with Regulation (EC) No 1907/2006 (the REACH Regulation). This information has not been reviewed or verified by the Agency or any other authority. The content is subject to change without prior notice.
Reproduction or further distribution of this information may be subject to copyright protection. Use of the information without obtaining the permission from the owner(s) of the respective information might violate the rights of the owner.
