Registration Dossier
Registration Dossier
Data platform availability banner - registered substances factsheets
Please be aware that this old REACH registration data factsheet is no longer maintained; it remains frozen as of 19th May 2023.
The new ECHA CHEM database has been released by ECHA, and it now contains all REACH registration data. There are more details on the transition of ECHA's published data to ECHA CHEM here.
Diss Factsheets
Use of this information is subject to copyright laws and may require the permission of the owner of the information, as described in the ECHA Legal Notice.
EC number: 231-867-5 | CAS number: 7772-98-7
- Life Cycle description
- Uses advised against
- Endpoint summary
- Appearance / physical state / colour
- Melting point / freezing point
- Boiling point
- Density
- Particle size distribution (Granulometry)
- Vapour pressure
- Partition coefficient
- Water solubility
- Solubility in organic solvents / fat solubility
- Surface tension
- Flash point
- Auto flammability
- Flammability
- Explosiveness
- Oxidising properties
- Oxidation reduction potential
- Stability in organic solvents and identity of relevant degradation products
- Storage stability and reactivity towards container material
- Stability: thermal, sunlight, metals
- pH
- Dissociation constant
- Viscosity
- Additional physico-chemical information
- Additional physico-chemical properties of nanomaterials
- Nanomaterial agglomeration / aggregation
- Nanomaterial crystalline phase
- Nanomaterial crystallite and grain size
- Nanomaterial aspect ratio / shape
- Nanomaterial specific surface area
- Nanomaterial Zeta potential
- Nanomaterial surface chemistry
- Nanomaterial dustiness
- Nanomaterial porosity
- Nanomaterial pour density
- Nanomaterial photocatalytic activity
- Nanomaterial radical formation potential
- Nanomaterial catalytic activity
- Endpoint summary
- Stability
- Biodegradation
- Bioaccumulation
- Transport and distribution
- Environmental data
- Additional information on environmental fate and behaviour
- Ecotoxicological Summary
- Aquatic toxicity
- Endpoint summary
- Short-term toxicity to fish
- Long-term toxicity to fish
- Short-term toxicity to aquatic invertebrates
- Long-term toxicity to aquatic invertebrates
- Toxicity to aquatic algae and cyanobacteria
- Toxicity to aquatic plants other than algae
- Toxicity to microorganisms
- Endocrine disrupter testing in aquatic vertebrates – in vivo
- Toxicity to other aquatic organisms
- Sediment toxicity
- Terrestrial toxicity
- Biological effects monitoring
- Biotransformation and kinetics
- Additional ecotoxological information
- Toxicological Summary
- Toxicokinetics, metabolism and distribution
- Acute Toxicity
- Irritation / corrosion
- Sensitisation
- Repeated dose toxicity
- Genetic toxicity
- Carcinogenicity
- Toxicity to reproduction
- Specific investigations
- Exposure related observations in humans
- Toxic effects on livestock and pets
- Additional toxicological data
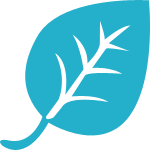
Endpoint summary
Administrative data
Description of key information
Environmental fate of sodium thiosulfate
Upon dissolution in environmental medium, sodium thiosulfate dissociates into the respective ions, i.e. thiosulfate anions(S2O32-) and sodium cations. Sodium is an abundant element, very soluble and occurs as monovalent cation under environmental conditions. Conclusively, sodium cations become part of the global sodium cycle.
Thiosulfates though naturally present in the environment are not stable under typical environmental conditions. Environmental transformation reactions include oxidation and reduction depending on the environmental conditions. Thiosulfates become ultimately part of the natural sulfur cycle resulting in sulfides and sulfates - indistinguishable from natural sulfur reservoirs.
Abiotic degradation: Photolysis, hydrolysis and photodegradation are not relevant as sodium thiosulfate dissociates rapidly and is transformed e.g. by oxidation, reduction, speciation, precipitation in environmental solutions.
Biotic degradation: The substance is an inorganic compound and is not subject to biodegradation.
Bioaccumulation: Sodium thiosulfate cannot bioaccumulate as it dissociates into thiosulfate anions and sodium cations upon release into the environment. Sodium ions are essential for plant and animal metabolism, do not bioaccumulate and are subject to homeostatic control. Thiosulfate anions are unstable under environmentally relevant conditions, are rapidly transformed into other sulfur species and ultimately become part of the global sulfur cycle. Sulfur is essential as structural component and on a metabolic level and does not bioaccumulate. Thus, bioaccumulation of sodium thiosulfate is not expected and biomagnification and a significant transfer in the food chain can be excluded.
Distribution: The fate of sodium thiosulfate is determined by the fate of its released ions upon dissolution, i.e. thiosulfate anions and sodium cations. Sodium is very soluble and occurs as monovalent cation that, under environmental conditions, tends to remain in the dissolved form once in solution. Sodium is an abundant element, however its mobility in aquatic and terrestrial ecosystems may be limited by its adsorption on clay minerals with high cation-exchange capacities. Sodium is an important element in the biosphere and is essential to human life (Salminen et al. 2005). Conclusively, sodium cations become part of the global sodium cycle.
Reliable baseline levels of sodium in 743 pristine water/sediment samples collected across Europe were determined based on the FOREGS Geochemical Baseline Mapping Programme (Salminen et al. 2005), yielding representative median sodium concentrations of 6676.72 mg/kg and 6.76 mg/L in sediment and stream water, respectively. Regarding the partitioning of sodium in the water column, stream water/sediment partition coefficients range from 78 L/kg (5thpercentile) to 15601 L/kg (95thpercentile) with a median logKp(solids-water in sediment) of 2.89 for sodium.
Based on the available literature data, thiosulfate anions are unstable under environmentally relevant conditions and are readily oxidized to sulfate or reduced to sulfide.Thus, any quantitatively relevant adsorption onto soil, sediments or suspended matter for sodium thiosulfate as well as its dissociation products is not to be expected.However, high-quality data are available on sulfur partitioning in aquatic ecosystems based on the FOREGS Geochemical Baseline Mapping Programme and additional supporting studies on sulfur partitioning on soils and marine sediments, yielding a log Kp(solids-water in freshwater sediment) of 2.02 L/kg (sulfur, n = 750), a log Kp(solids-water in marine sediment) of 1.58 L/kg (sulfur, n = 2) and a log Kp(solids-water in soil) of 1.64 L/kg (sulfur, n = 25).
Additional information
Abiotic and biotic processes determining the fate of sulfites and thiosulfates in the environment
Upon contact with water, salts of sulfur oxyacids including sodium thiosulfate dissociate into sulfur oxyacid anions and the respective counterions. Only the properties of the thiosulfate anions are considered a relevant determinant of environmental toxicity since respective counter ions, i.e. ammonium, calcium, magnesium, sodium or potassium cations, are not assumed to affect the toxicity.
Regarding the fate of thiosulfates and dithionites in the environment, both H2S2O3and H2S2O4 are strong acids and are dissociated under environmentally relevant conditions with their thiosulfate(S2O32-)and dithionite(S2O42-) anions being unstable and readily oxidized toSO42-(Lindsay, 1979). Similarly, sulfurous acid (H2SO3)and its salts (i.e. sulfites) are generally considered as unstable in soils due to quick abiotic turnover to SO42-(oxidation) or by microbial action (see below).
Therefore, metal thiosulfates are generally not expected to persist under oxidising conditions, e.g. in surface waters or topsoils.Environmental transformation reactions include oxidation and reduction depending on the environmental conditions. Thiosulfates become ultimately part of the natural sulfur cycle resulting in sulfides and sulfates - indistinguishable from natural sulfur reservoirs.
The environmental fate and transformation of sodium thiosulfate and its dissociation products depend on the environmental conditions as described in the following:
All sulfite, hydrogensulfite and metabisulfite substances are highly soluble in water, establishing upon dissolution an equilibrium that depends on solution pH as follows (Wiberg et al., 1996; Lide, 2007):
1. SO2+ H2O ⇌ H2SO3
2. H2SO3 ⇌ H++ HSO3- pka1: 1.85
3. HSO3- ⇌ H++ SO32- pka2: 7.20
4. 2 HSO3- ⇌ H2O + S2O52-
In acidic solutions, sulfites and hydrogen sulfites may release SO2but this is not likely to occur under e.g. normal natural environmental conditions.
5. SO32-+ 2 H3O+⇌SO2+ 3 H2O
Under oxidising conditions, e.g., in surface waters, sulfite is rapidly oxidized to sulfate catalytically by (air) oxygen or by microbial action. A half-life of 77 hour was measured in deionized water, already suggesting substantial abiotic degradation.
6. 2 SO32-+ O2⇌2 SO42-(in presence of oxygen)
The reaction is accompanied with consumption of dissolved oxygen. Thus, observed toxic effects to aquatic organisms may be indirect effects, i.e. caused by lack of oxygen.
Thiosulfate:
The structure of the thiosulfate ion (S2O32-) is comparable to the sulfate ion with one oxygen atom replaced by a sulfur atom. It is considered to be metastable and has only moderate reducing properties (Cotton et al., 1999). Thus, thiosulfate is an oxyanion of sulfur, is the respective anion of the strong thiosulfuric acid and the only species relevant upon dissolution in an aqueous medium under environmental conditions:
7. H2S2O3+ H2O⇌HS2O3-+ H3O+ pka1: 0.6
8. HS2O3-+ H2O⇌S2O32- + H3O+ pka2: 1.74
Thiosulfates occur naturally and are produced by certain biochemical processes. Thiosulfates are stable only in neutral or alkaline solutions, but not in acidic (physiological) solutions, due to decomposition to sulfite and sulfur, the sulfite being dehydrated to SO2:
9. S2O32-(aq)⇌SO32-(aq) + S (s)
10. S2O32-(aq) + 2 H3O+(aq)⇌SO2(g) + S (s) + H2O
Thus, in contrast to sulfite, the disproportionation of thiosulfates occurs under acid but not under alkaline conditions. Regarding the fate of thiosulfates, H2S2O3is a strong acid and is dissociated under environmentally relevant conditions, with thiosulfate (S2O32-) anions being unstable and readily oxidized to SO42-(Lindsay, 1979). Under normal environmental conditions, a break down to sulfite and a subsequent oxidation to sulfate may be anticipated.
The presence of metal cations in the environment, such as copper, iron and manganese, accelerates the oxidation rate. The most stable and predominant sulfur form in freshwater and in all but highly reduced environments is sulfate (SO42-).
In addition to the abiotic processes, microbial oxidation of sulfur compounds is an energetically favourable reaction carried out by a wide range of organisms, i.e. sulfur oxidizing microorganisms (SOM), which may oxidize reduced sulfur species including sulfite (SO32-), thiosulfate (S2O32-), elemental sulfur (S) and sulfide (HS-), resulting in ultimate transformation into sulfate (SO42-) (Simon and Kroneck, 2013). Therefore, regarding the fate of sulfites and thiosulfates in the environment, thiosulfuric acid (H2S2O3) and its salts (i.e. thiosulfates) are generally considered as unstable in soils due to quick abiotic turnover to SO42-(oxidation) or by microbial action.
Under anoxic conditions, the resulting sulfate will be readily reduced to sulfide by sulfate-reducing bacteria (SRM) which are common in anaerobic environments. However, also other substrates, e.g. dithionite (S2O42-), thiosulfates (S2O32-) or sulfite (SO32-) may be used in this anaerobic process, ultimately resulting in reduction to sulfide (H2S). Available data show very low levels of free sulfide in pore waters of marine and freshwater sediments, i.e. levels of < 1 µM free sulfide in sediments of the Odder River and Brabrand Lake (Denmark) (Jørgensen, 1990), presumably due to rapid formation of insoluble metal sulfides. Therefore, the reduction of sulfates, sulfites and thiosulfates to sulfides may ultimately result in formation of solid-phase minerals and metal sulfides of very low bioavailability/solubility, e.g. FeS, ZnS, PbS and CdS (Lindsay, 1979; OECD SIDS, 2012). Nevertheless, metal sulfides are not expected to persist under oxidising conditions, e.g. in surface water or topsoil.
Additionally, a significant set of microorganisms is able to grow by disproportionation of thiosulfate, sulfite or elemental sulfur, ultimately resulting in sulfate and sulfide (Simon and Kroneck, 2013 and references therein; Janssen et al. 1996, Bak and Cypionka, 1987):
11. 4 SO32-+ H+⇌3 SO42-+ HS-
12. S2O32-+ H2O⇌SO42-+ HS-+ H+
13. 4 S0+ 4 H2O⇌SO42-+ 3 HS-+ 5 H+
In presence of metal ions, e.g. ferrous iron, the resulting sulfide is expected to precipitate rapidly as insoluble metal sulfides, e.g. FeS, resulting in very low dissolved sulfide levels (Finster et al. 1998).
Water:
In aqueous systems, a quick turnover of S2O32- to SO42- and/or sulfide (HS-) is expected.
Data on thiosulfate (S2O32-) transformation processes are available from a study performed by Findlay and Kamyshny (2017): When amended to anoxic freshwaters at realistic environmental concentrations (approx. 125 µM, added as sodium salt), thiosulfate (S2O32-) was consumed with no lag time at an initial rate of 3.0 µM/h in a non-sterilized experiment with > 95% removal within 96h.
In addition, data are available on thiosulfate transformation in batch experiments using activated sludge: In a study performed by Tarekegn et al. (2016), thiosulfate concentrations dropped from approx. 0.29 g/L to 0.02 g/L within a period of 9 days, corresponding to a > 93 % reduction in thiosulfate concentrations. Thiosulfates were efficiently removed under anaerobic conditions with an observed increase in sulfate and H2S concentrations. However, no radiotracers were used in the study, the media was poorly described and no pH monitoring was performed and results should therefore be treated with caution.
In sum, the fate of thiosulfates in aqueous media is accurately described by transformation including reductive and oxidative processes and disproportionation with ultimate transformation to H2S and SO42-.
Sediment:
Zopfi et al. (2004) reported very low thiosulfate concentrations in Black Sea sediments (< 3 µM S2O32-), presumably attributable to thiosulfate-consuming bacteria. Regarding the transformation of thiosulfate in Black sea sediment under environmental relevant conditions, thiosulfate added at very high concentrations of 82 µM and 21 µM S2O32-showed high disappearance rates of 42 and 8.5 µM/h, respectively. Additional supporting data on thiosulfate (S2O32-) transformation processes are available from a study performed by Findlay and Kamyshny (2017): S2O32-was amended to the sediments at an environmentally relevant concentration of 140 µM (added as sodium salt) and experiments were run in absence of oxygen. S2O32-was consumed fast with very low thiosulfate concentrations (approx. < 5 µM) observed after one week.
In a study performed by Jørgensen et al. (1990), time-course experiments with different sediment slurries using 35S-thiosulfate radiotracers at environmentally relevant concentrations (80-125 µM S2O32-) were performed in order to investigate potential environmental transformations in freshwater sediments. Sediments were derived from two freshwater localities, i.e. the Odder River and Brabrand Lake (2-3 m average depth) near Aarhus (Denmark). In both sediments investigated, i.e. freshwater sediments from the Odder river and Lake Brabrand, S2O32-was consumed at a constant rate and depleted after 5-6 h, indicating rapid transformation processes.
In sum, thiosulfate is of limited stability in sediments.
Soil:
In addition to the abiotic processes, microbial oxidation of sulfur compounds is an energetically favourable reaction carried out by a wide range of organisms, i.e. sulfur oxidizing microorganisms (SOM), which may oxidize reduced sulfur species including thiosulfate (S2O32-), sulfite (SO32-), elemental sulfur (S) and sulfide (HS-), resulting in ultimate transformation into sulfate (SO42-, Simon and Kroneck, 2013):
S2O32-⇌HSO3-/ SO2⇌SO32-→ SO42-
Accordingly, data on thiosulfate stability in soil is available from a study performed by Barbosa-Jefferson et al. (1998) using four top soils (0-15 cm) from major arable areas in Britain. S2O32-added to four arable soils at high concentrations showed rapid and complete transformation primarily to sulfate (SO42-).
In sum, the fate of thiosulfates in soil is accurately described by predominantly oxidative pathways (either biotic or abiotic), ultimately leading to transformation of thiosulfate (S2O32-) into sulfate (SO42-), therefore entering the sulfur cycle (see Fig. 1, Section 5.6: Additional information on environmental fate and behaviour: S2O3_Literature Data_Fate and transformation of thiosulfates in soil).
Environmental sulfur/sulfate concentrations:
A total of 750 stream water samples were processed in the FOREGS-program to determine typical sulfur stream water concentrations (Salminen et al. 2005).The FOREGS Geochemical Baseline Mapping Programme’s main objective is to provide high quality, multi-purpose homogeneous environmental geochemical baseline data for Europe. Based on the FOREGS dataset, sulfate concentrations in European stream waters may range from 1.18 mg/L (5thpercentile) to 163.8 mg/L (95thpercentile) with a median of 16.9 mg/L. Total sulfur concentrations of streamwater sediments are magnitudes higher with 50thand 95thpercentiles of 508 and 2816 mg sulfate/L, respectively.Regarding the partitioning of sulfur in the water column, stream water/sediment partition coefficients range from 1.28 L/kg to 15729.3 L/kg with a median logKp(solids-water in sediment) of 2.02 for sulfur (with concentrations of the water based on measured sulfate as the predominant sulfur species and total sulfur of the sediment).
Regarding background soil concentrations, baseline sulfur levels in topsoil (0-25 cm) range from < 50 mg/kg to 6518 mg/kg sulfur with 5th, 50thand 95thpercentiles of 75 mg/kg, 222 mg/kg and 645 mg/kg sulfur, respectively. Taking into account the high quality and representativeness of the dataset, the 95thpercentile of 645 mg/kg sulfur can be regarded as typical background sulfur concentrations of topsoil in EU countries. In addition, ambient sulfur concentrations of agricultural and grazing land soil are available. Sulfur levels of agricultural soil range from < 5.00 to 68226.3 mg/kg sulfur with 5thand 95th percentiles of 59.7 and 783.9 mg/kg sulfur, respectively. In grazing land, soil sulfur concentrations range from < 5.00 to 98189.7 mg/kg with 5thand 95thpercentiles of 67.3 and 1288.6 mg/kg sulfur, respectively.Taking into account the high quality and representativeness of the data set, the 95thpercentile of 783.91 mg/kg can be regarded as representative background concentration for sulfur in European agricultural soils and the 95thpercentile of 645 mg/kg can be regarded as representative background concentration for sulfur in European grazing land soils.
References:
Finster, K., Liesack, W., & Thamdrup, B. O. (1998). Elemental sulfur and thiosulfate disproportionation by Desulfocapsa sulfoexigens sp. nov., a new anaerobic bacterium isolated from marine surface sediment. Applied and Environmental Microbiology, 64(1), 119-125.
Lide, D. R. (2007). Handbook of Chemistry and Physics, volume 88th edition.CRC Press,22(24), 154.
Wiberg, N., & Dehnicke, K. (1996). Hollemann-Wiberg, Lehrbuch der Anorganischen Chemie.Angewandte Chemie-German Edition, 108(21), 2696.
Information on Registered Substances comes from registration dossiers which have been assigned a registration number. The assignment of a registration number does however not guarantee that the information in the dossier is correct or that the dossier is compliant with Regulation (EC) No 1907/2006 (the REACH Regulation). This information has not been reviewed or verified by the Agency or any other authority. The content is subject to change without prior notice.
Reproduction or further distribution of this information may be subject to copyright protection. Use of the information without obtaining the permission from the owner(s) of the respective information might violate the rights of the owner.
