Registration Dossier
Registration Dossier
Data platform availability banner - registered substances factsheets
Please be aware that this old REACH registration data factsheet is no longer maintained; it remains frozen as of 19th May 2023.
The new ECHA CHEM database has been released by ECHA, and it now contains all REACH registration data. There are more details on the transition of ECHA's published data to ECHA CHEM here.
Diss Factsheets
Use of this information is subject to copyright laws and may require the permission of the owner of the information, as described in the ECHA Legal Notice.
EC number: 231-442-4 | CAS number: 7553-56-2
- Life Cycle description
- Uses advised against
- Endpoint summary
- Appearance / physical state / colour
- Melting point / freezing point
- Boiling point
- Density
- Particle size distribution (Granulometry)
- Vapour pressure
- Partition coefficient
- Water solubility
- Solubility in organic solvents / fat solubility
- Surface tension
- Flash point
- Auto flammability
- Flammability
- Explosiveness
- Oxidising properties
- Oxidation reduction potential
- Stability in organic solvents and identity of relevant degradation products
- Storage stability and reactivity towards container material
- Stability: thermal, sunlight, metals
- pH
- Dissociation constant
- Viscosity
- Additional physico-chemical information
- Additional physico-chemical properties of nanomaterials
- Nanomaterial agglomeration / aggregation
- Nanomaterial crystalline phase
- Nanomaterial crystallite and grain size
- Nanomaterial aspect ratio / shape
- Nanomaterial specific surface area
- Nanomaterial Zeta potential
- Nanomaterial surface chemistry
- Nanomaterial dustiness
- Nanomaterial porosity
- Nanomaterial pour density
- Nanomaterial photocatalytic activity
- Nanomaterial radical formation potential
- Nanomaterial catalytic activity
- Endpoint summary
- Stability
- Biodegradation
- Bioaccumulation
- Transport and distribution
- Environmental data
- Additional information on environmental fate and behaviour
- Ecotoxicological Summary
- Aquatic toxicity
- Endpoint summary
- Short-term toxicity to fish
- Long-term toxicity to fish
- Short-term toxicity to aquatic invertebrates
- Long-term toxicity to aquatic invertebrates
- Toxicity to aquatic algae and cyanobacteria
- Toxicity to aquatic plants other than algae
- Toxicity to microorganisms
- Endocrine disrupter testing in aquatic vertebrates – in vivo
- Toxicity to other aquatic organisms
- Sediment toxicity
- Terrestrial toxicity
- Biological effects monitoring
- Biotransformation and kinetics
- Additional ecotoxological information
- Toxicological Summary
- Toxicokinetics, metabolism and distribution
- Acute Toxicity
- Irritation / corrosion
- Sensitisation
- Repeated dose toxicity
- Genetic toxicity
- Carcinogenicity
- Toxicity to reproduction
- Specific investigations
- Exposure related observations in humans
- Toxic effects on livestock and pets
- Additional toxicological data
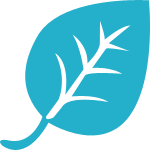
Endpoint summary
Administrative data
Description of key information
Additional information
Iodine is a naturally occurring element and is ubiquitous in all compartments of the environment. In 1984 Fabryka-Martin developed an extended version of the model for the distribution of iodine in the environmental compartments (Fabryka-Martin, 1984), on the basis of a steady-state model for the geochemical lifecycle of iodine by Kocher (Kocher, 1981). In both models complete mixing of the compartments is assumed. Major changes are the inclusion of the igneous rocks and sediments (lithosphere) compartments which are not in active exchange with the ocean, as well as the assumption that these compartments are in exchange with deep groundwater and the atmosphere only. Within the model about 95% of the global iodine inventory is assumed to be stored in the lithosphere with a mean residence time of more than 1E+08 years. An additional fraction of about 4% of total iodine is expected to be bound in recent ocean sediment. The mean residence time for this compartment is estimated to be about four million years. The remaining iodine (approx. 1%) is available for the lifecycle with the ocean considered to be the major reservoir.
In the Fabryka-Martin model significant pathways are the migration of iodine from the lithosphere to the atmosphere and the hydrosphere (deep groundwater) by volcanic activities, combustion of fossil fuels and the weathering of rocks. Furthermore the exchange between recent ocean sediments and the deep-ocean as well as inner-oceanic mixing are important transport processes within the marine compartment. In addition, iodine is transferred from the freshwater segment to the ocean. The mixed-layer of the ocean is in constant exchange with the marine atmosphere by evaporation, sea spray, biogenic emissions plus dry and wet deposition in return. By different atmospheric processes iodine is distributed and transported to the pedosphere. The atmospheric and pedospheric exchange occurs by dry and wet deposition and by evaporation of iodine as such or as biogenic emission. Finally, the biosphere takes up iodine from hydrosphere and pedosphere and loses iodine to the atmosphere and pedosphere by evaporation, decomposition as well as excretion.
Rucklidge et al. set up a simplified model for estimating the speciation of iodine in the environment. While neglecting the massive influence of organic matter and microorganisms on the speciation of iodine, in general, the species can be assessed by prevalent Eh- and pH-values of the environment. In this context it is assumed that molecular iodine and iodide are major compounds under common environmental conditions (attached diagram) (Rucklidge, 1994). However, since iodine is a biophilic element, iodine forms organic compounds in all compartments which have to be considered in the geochemical lifecycle. Hypoiodous acid (HOI) is proposed to be a key species for this reactions in aqueous solutions (Truesdale, 1994; Baker, 2005). Furthermore iodinated VOCs are important for the transfer between the ocean and the marine atmosphere. Key substances are methyliodide (CH3I) and diiodomethane (CH2I2) which are emitted by algae and marine bacteria (Carpenter, 1999; Amachi, 2001).
Since methyliodide is also a metabolism product of terrestrial bacteria it is expected to be an important compound in the soil-air transfer (Amachi, 2001). In soil the behavior of iodine is influenced ,inter alia, by organic soil compounds (Johnson, 1980) as well as iron or aluminium hydroxides (Whitehead, 1973) which interact with iodine species and fix them. In addition, the mobility of iodine is affected by soil redox conditions. Findings of Ashworth et al. indicate that iodine is less mobile within oxic zones of soil and tends to accumulate in the transition zones between anoxic and oxic zones in the soil compartment (Ashworth, 2003; Ashworth, 2006). In case of soil saturation with iodine it is expected that additional iodine passes readily through the soil without being retained (Fuge, 1986).
In seawater the concentration of iodine ranges from 40µg/L to 65 µg/L with varying ratios of iodide and iodate depending on depth and geographical location. While at surface level the fraction of iodide is about 50%, the fraction of iodate increases with decreasing depth. At bottom level iodate is the dominating species (Hou, 1999). In strong anoxic environments, e.g. East Sea, iodide is prevalent (Wong, 1991).Elemental iodine as well as hypoiodous acid were not detected in seawater (Wong, 1991).
Major species for the iodine transfer between the ocean and the marine atmosphere are primary the iodinated volatile organic compounds methyliodide and diiodomethane with concentrations of 3 pptv, respectively 0.1 pptv for measurements at the Irish coast and elemental iodine by iodovolatilisation from macro-algae (Carpenter, 1999; Saiz-Lopez, 2004; Saiz-Lopez, 2006).
In the atmosphere the iodine concentration in the gas phase ranges from 25 pptv to 115 pptv at daytime and from 63 to 200 pptv at nighttime (Saiz-Lopez, 2004; Saiz-Lopez, 2006; Peters, 2005; Huang, 2010). In aerosols the average concentration of total soluble iodine is about 220 pmol/m3(air) with a distribution in ~ 90% soluble organic iodine (SOI), ~ 6% iodide and ~ 1% iodate (Gilfedder, 2008). In rain and snow the total soluble iodine concentration ranges from 5.2 nmol/L to 13.7 nmol/L (iodide: 1.8-4.1 nmol/L, iodate: 0.17-1.3 nmol/L, SOI: 1.34-8.7 nmol/L), respectively 0.9 nmol/L to 18.7 nmol/L (iodide: 0.31-5.7 nmol/L, iodate: <0.2-0.46 nmol/L, SOI: 0.09-12.6 nmol/L) for different sampling locations in the Northern and Southern Hemisphere (Gilfedder, 2008).
In rock-forming minerals the concentration of iodine is constantly very low (<0.02 - 1.2 mg/kg); similar concentrations were found in igneous rocks (<0.02 - 1.9 mg/kg). Higher concentrations were found in sediments (up to 200 µg/g). In soil the average concentration is assumed to be about 4-8 mg/kg (Fuge, 1986). As stated above, the speciation of iodine in soil is depending on several environmental factors, like content of organic matter, presence of Fe/Al hydroxides, pH, soil redox potential etc.
In freshwater the iodine concentration ranges from 0.1 to 18 µg/L with iodide being the major species (about 90%, FOREGS database).
References:
Amachi S, Kamagata Y, Kanagawa T, Muramatsu Y (2001). Bacteria mediate methylation of iodine in marine and terrestrial environments, Appl. Environ. Microbiol., 2718-2722.
Ashworth DJ, Shaw G, Butler AP, Ciciani L (2003). Soil transport and plant uptake of radio-iodine from near-surface groundwater, J Environ Radioactivity, 70, 99-114.
Ashworth DJ, Shaw G (2006). Effects of moisture content and redox potential on in situ Kd values for radioiodine in soil, Sci Total Environ, 359, 244-254.
Baker AR (2005). Marine Aerosol Iodine Chemistry: The Importance of Soluble Organic Iodine, Environ Chem, 2, 295-298.
Carpenter LJ, Sturges WT et al. (1999). Short-lived alkyl iodides and bromides at Mace Head, Ireland: Links to biogenic sources, J. Geophys. Res., 104, 1679– 1689.
Fabryka-Martin J, Bentley H, Elmore D, Airey PL (1984). Natural iodine-129 as an environmental tracer, Geochim Cosmochim Acta, 49, 337-347.
Fuge R, Johnson CC (1986). The geochemistry of iodine - a review, Environ Geochem Health, 8(2), 31-54.
Gilfedder BS, Lai SC, Petri M, Biester H, Hoffmann T (2008) Iodine speciation in rain, snow and aerosols, Atmos Chem Phys, 8, 6069 -6084.
Hou XL, Dahlgaard H, Rietz B et al. (1999). Determination of 129I in seawater and some environmental materials by neutron activation analysis, Analyst 124, 1109-1114.
Huang RJ, Seitz K, Buxmann J et al. (2010). In situ measurements of molecular iodine in the marine boundary layer: the link to macroalgae and the implications for O3, IO, OIO and NOx, Atmos Chem Phys, 10, 4823-4833.
Johnson CC (1980). The geochemistry of iodine and a preliminary investigation into its potential use as a pathfinder element in geochemical exploration, Ph.D. thesis, University College of Wales, Aberystwyth.
Kocher DC (1981). A dynamic model of the global iodine cycle and estimation of dose to the world population from releases of Iodine-129 to the environment, Envt Intl, 5, 15-31.
Peters C, Pechtl S, Stutz J et al. (2005). Reactive and organic halogen species in three different European coastal environments, Atmos Chem Phys, 5, 3357-3375, doi: 10.5194/acp-5-3357-2005.
Rucklidge J, Kilius L, Fuge R (1994). 129-Iodine in moss down-wind from the Sellafield nuclear fuel reprocessing plant, Nuclear Instruments and Methods in Physics Research B 92, 417-420.
Saiz-Lopez A, Plane JMC (2004). Novel iodine chemistry in the marine boundary layer, Geophys Res Lett, 31, L04112, doi: 10.1029/2003GL019215.
Saiz-Lopez A, Plane JMC, McFiggans G et al. (2006). Modelling molecular iodine emissions in a coastal marine environment: the link to new particle formation, Atmos Chem Phys, 6, 883-895, doi: 10.5194/acp-6-886-2006.
Truesdale VW, Luther GW (1995). Molecular iodine reduction by natural and model organic substances in seawater, Aq. Geochem., 1, 89-104.
Whitehead DC (1973). Studies on iodine in British soils, J Soil Sci, 24, 260-270.
Wong GTF (1991). The marine geochemistry of iodine, Reviews Aquatic Sci, 4(1), 45-73.
Information on Registered Substances comes from registration dossiers which have been assigned a registration number. The assignment of a registration number does however not guarantee that the information in the dossier is correct or that the dossier is compliant with Regulation (EC) No 1907/2006 (the REACH Regulation). This information has not been reviewed or verified by the Agency or any other authority. The content is subject to change without prior notice.
Reproduction or further distribution of this information may be subject to copyright protection. Use of the information without obtaining the permission from the owner(s) of the respective information might violate the rights of the owner.
