Registration Dossier
Registration Dossier
Data platform availability banner - registered substances factsheets
Please be aware that this old REACH registration data factsheet is no longer maintained; it remains frozen as of 19th May 2023.
The new ECHA CHEM database has been released by ECHA, and it now contains all REACH registration data. There are more details on the transition of ECHA's published data to ECHA CHEM here.
Diss Factsheets
Use of this information is subject to copyright laws and may require the permission of the owner of the information, as described in the ECHA Legal Notice.
EC number: 231-442-4 | CAS number: 7553-56-2
- Life Cycle description
- Uses advised against
- Endpoint summary
- Appearance / physical state / colour
- Melting point / freezing point
- Boiling point
- Density
- Particle size distribution (Granulometry)
- Vapour pressure
- Partition coefficient
- Water solubility
- Solubility in organic solvents / fat solubility
- Surface tension
- Flash point
- Auto flammability
- Flammability
- Explosiveness
- Oxidising properties
- Oxidation reduction potential
- Stability in organic solvents and identity of relevant degradation products
- Storage stability and reactivity towards container material
- Stability: thermal, sunlight, metals
- pH
- Dissociation constant
- Viscosity
- Additional physico-chemical information
- Additional physico-chemical properties of nanomaterials
- Nanomaterial agglomeration / aggregation
- Nanomaterial crystalline phase
- Nanomaterial crystallite and grain size
- Nanomaterial aspect ratio / shape
- Nanomaterial specific surface area
- Nanomaterial Zeta potential
- Nanomaterial surface chemistry
- Nanomaterial dustiness
- Nanomaterial porosity
- Nanomaterial pour density
- Nanomaterial photocatalytic activity
- Nanomaterial radical formation potential
- Nanomaterial catalytic activity
- Endpoint summary
- Stability
- Biodegradation
- Bioaccumulation
- Transport and distribution
- Environmental data
- Additional information on environmental fate and behaviour
- Ecotoxicological Summary
- Aquatic toxicity
- Endpoint summary
- Short-term toxicity to fish
- Long-term toxicity to fish
- Short-term toxicity to aquatic invertebrates
- Long-term toxicity to aquatic invertebrates
- Toxicity to aquatic algae and cyanobacteria
- Toxicity to aquatic plants other than algae
- Toxicity to microorganisms
- Endocrine disrupter testing in aquatic vertebrates – in vivo
- Toxicity to other aquatic organisms
- Sediment toxicity
- Terrestrial toxicity
- Biological effects monitoring
- Biotransformation and kinetics
- Additional ecotoxological information
- Toxicological Summary
- Toxicokinetics, metabolism and distribution
- Acute Toxicity
- Irritation / corrosion
- Sensitisation
- Repeated dose toxicity
- Genetic toxicity
- Carcinogenicity
- Toxicity to reproduction
- Specific investigations
- Exposure related observations in humans
- Toxic effects on livestock and pets
- Additional toxicological data
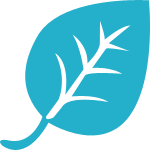
Adsorption / desorption
Administrative data
Link to relevant study record(s)
- Endpoint:
- adsorption / desorption, other
- Remarks:
- adsorption
- Type of information:
- experimental study
- Adequacy of study:
- key study
- Reliability:
- 2 (reliable with restrictions)
- Rationale for reliability incl. deficiencies:
- other: Study well documented, meets generally accepted scientific principles, acceptable for assessment
- Justification for type of information:
- This data which uses NaI as the test substance, is considered relevant for read across to iodine as iodide is the major existent species of iodine in soil which is available for interactions with soil components.
- Qualifier:
- no guideline followed
- Principles of method if other than guideline:
- Experimental settings give the opportunity to determine Kd values under more realistic soil moisture contents as well as time-dependent changes in the system (e.g. changes in redox potential)
- GLP compliance:
- no
- Type of method:
- other: mini-column approach
- Media:
- soil
- Radiolabelling:
- yes
- Test temperature:
- Tests were performed in a controlled environment room with 18 hours daytime at 20 °C and 6 hours nighttime at 15 °C. Relative humidity was controlled at 70%.
- Details on study design: HPLC method:
- As iodine is an inorganic compound and is known to form complex compunds with minerals and clay adsorption testing according to OECD guideline 121 is not applicable. It can be expected that the iodine would interact with the stationary phase of the HPLC column.
- Analytical monitoring:
- yes
- Details on sampling:
- - Sampling interval: on days 1, 4 , 7, 10, 14, 21, 28, 35, 42 and 49 a 3 mL soil solution sample was removed
After the removal of the samples, the columns were re-weighted and deionised water was added to restore their initial mass. As only the removed amount of water was refilled it can be assumed that evaporisation losses were minimized. - Details on matrix:
- COLLECTION AND STORAGE
- Geographic location: Silwood Park (Ascot, UK)
- Collection procedures: no information available
- Sampling depth (cm): 0-20 cm
- Storage conditions: three samples were gravimetrically adjusted to 25 % moisture content, three samples were adjusted to 40 % moisture content. Both adjustments were carried out by adding deionised water.
- Storage length: no information available
- Soil preparation (e.g.: 2 mm sieved; air dried etc.): 2mm2 sieved
PROPERTIES
- Soil texture
- % sand: 75
- % silt: 20
- % clay: 5
- Soil taxonomic classification: sandy loam topsoil
- Soil classification system: no information available
- Soil series: Wicks series
- Soil order: no information available
- pH: 4.3
- Organic carbon (%): 4.7
- CEC (meq/100 g): no information available
- Carbonate as CaCO3: no information available
- Insoluble carbonates (%): no information available
- Extractable Cations (Ca, Mg, Na, K, H) (MEQ/100 g): no information available
- Special chemical/mineralogical features: no information available
- Clay fraction mineralogy: no information available
- Moisture at 1/3 atm (%): no information available
- Bulk density (g/cm3): soils were packed in column to produced equivalent to dry bulk density of 1.3 g/cm3
- Biomass (e.g. in mg microbial C/100 mg, CFU or other): no information available - Details on test conditions:
- At first 1 mL I-125 containing solution (1.5 kBq) was added to each soil sample and afterwards manually mixed for homogenisation of the sample. Then tree probes were taken from each sample and the specific initial activity were determined (less than 10 % variation). The prepared soils were packed into the columns to a depth of 12.5 cm to produce an equivalent to a dry bulk density of 1.3 g/cm3.
For the 40% moisture experiments saturation was monitored by existence of standing water at soil surface. Additionally, the columns were sealed with a rubber bung to limit the access of atmospheric oxygen since the aim of these experiments was produce anoxic conditions. All probes were stored in a controlled environmental room at 70% relative humidity and were exposed to light by an artificial day-night cycle (18 h, 20 °C/ 6 h , 15 °C). - Type:
- Kd
- Value:
- >= 0.13 - <= 7.7 L/kg
- Temp.:
- 20 °C
- % Org. carbon:
- ca. 4.7
- Remarks on result:
- other: in dry (oxic) soils; simulated day/night-cycle with different temperatures
- Type:
- Kd
- Value:
- >= 0.19 - <= 2.3 L/kg
- Temp.:
- 20 °C
- % Org. carbon:
- ca. 4.7
- Remarks on result:
- other: in saturated (anoxic) soils; simulated day/night-cycle with different temperatures
- Transformation products:
- not measured
- Conclusions:
- The presented mini-column approach of Ashworth is an alternative to the batch equilibrium method as long-term behaviour of substances under more realistic soil conditions and time-dependend changes (e.g. redox potential) can be observed. Although it is no guideline study, it is performed and documented in accordance to generally accepted scientific practice. Thus, it can be considered adequate for the chemical risk assessment.
According to Annex XI.1.1.2 data shall be considered as equivalent to data generated by the corresponding test methods referred to in Article 12 (3) if they are adequate for the purpose of classification and labelling and/ or risk assessment, the key parameters forseen to be investigated in the corresponding test methods according to Art. 13(3) adequate and reliable covered, the exposure duration is comparable to or longer than the corresponding test methods referred to in Art. 13 (3) (if exposure duration is relevant) and an adequate and reliable documentation of the study is provided.
As Iodine is an inorganic element and is known for forming complex compounds with minerals and clay adsorption testing in accordance to OECD guideline 121 is not applicable. It can be expected that the iodine would interact with the stationary phase of the HPLC column.
The presented data is a new approach for long term (50 days) determination of Kd values under more realistic soil moisture conditions and a system in which time-dependent processes such as changes in redox potential can take place. - Executive summary:
Ashworth et al. used a new mini-column approach to determine the Kd value of iodine in soil. They took topsoil samples and added a certain amount of I-125 to each sample. Two series with different soil conditions (dry (oxic) and saturated (anoxic)) were established and the Kd value as well as the redox potential of the soil on day 1, 4, 7, 10, 14, 21, 28, 35, 42 and 49 measured. They were able to obtain a Kd value from 0.13 to 7.7 L/kg for oxic and from 0.19 to 2.3 L/kg for anoxic soil conditions.
Additionally, Ashworth et al. performed a single batch equilibrium experiment for the same soil sample for comparative purposes. They used several I-125 treatments to establish different equilibria and determined a Kd value of 2.5 L/kg.
From the long-term observations of the mini-column experiment it is identifiable that the Kd value increases over the course of the test reaching different saturation levels at the end of the test. The parallel analysis of the sulphate and nitrate concentrations as indicator for the soil redox status as well as the direct measurement of the soil redox potential showed that similar redox conditions were prevalent over the first 30 days of the experiment for both test series. Afterwards the redox potential for the saturated columns dropped to -150 mV and the nitrate concentration decreased by around 60% from the start to the end of the experiment.
Based on the results of this report the mini-column approach can be considered as reasonable extension of the current test regime as it allows long-term observations under more realistic soil conditions as well as time-dependend processes. The observed results for iodine seem to be consistent with geometric mean compendia values and indicates that iodine within oxic environments is less mobile and, probably, less bio-available than in anoxic environments.
- Endpoint:
- adsorption / desorption: screening
- Type of information:
- read-across from supporting substance (structural analogue or surrogate)
- Adequacy of study:
- supporting study
- Reliability:
- 2 (reliable with restrictions)
- Rationale for reliability incl. deficiencies:
- study well documented, meets generally accepted scientific principles, acceptable for assessment
- Qualifier:
- no guideline followed
- Principles of method if other than guideline:
- Adsorption–desorption of iodine in two forms, viz., iodide (I−) and iodate (IO3-), in three types of soil were investigated.
- GLP compliance:
- not specified
- Media:
- soil
- Radiolabelling:
- no
- Test temperature:
- 25°C
- Analytical monitoring:
- yes
- Details on matrix:
- The representative soils used were collected from three different sites in Zhejiang Province, China. The red soil (REQ) developed on Quaternary red earths (clayey, kaolintic thermic plinthite Aquult) was collected from the interval of Longyou county. The Inceptisol (IS) developed from fluvio-aquic soil matrix (clayey) and the alluvial soil (AS) developed from coastal saline soil matrix (Fluviomarine yellow loamy soil) were collected from the old vegetable production area in the city suburbs of Hangzhou and Jiaxing, respectively.
These soils were air-dried, ground, and screened through a 2-mm sieve prior to use. The basic characteristics of soils used in the experiment are listed as follows: pH (1:2.5 suspension of soil and water) was 4.56, 5.83, and 7.34; the organic matter was 20.8, 40.9, and 15.8 g/kg; and total iodine was 0.68, 2.21, and 4.22 mg /kg for REQ, IS, and AS, respectively. The corresponding value of CEC (at solution pH of 7.0) was 16.2, 19.3, and 11.6 cmol /kg; free Fe oxide was 3.98%, 1.55%, and 1.64% and free Al oxide was 6.35%, 1.89%, and 2.21%. The distribution (%) of particle size <0.002, 0.002–0.02, and 0.02–2.0 mm was 40.5, 49.4, and 10.1 for REQ, 38.9, 45.5, and 15.6 for IS, and 16.8, 45.4, and 37.8 for AS, respectively. - Details on test conditions:
- - Adsorption of Iodine:
Twenty samples each weighing 1.0 g (air-dried) from each type of soil were placed into 25-mL polypropylene centrifuge tubes. Iodine solutions with different concentrations, viz., 0, 1, 2, 4, 6, 8, 10, 12, 16, and 20 mg I/L (through KI as well as KIO3) were prepared in 0.01 M CaCl2 solution. Then, 10 mL of solutions containing each of the iodine concentrations and species was added separately to each tube. The suspensions were shaken at 160 rpm for 40 h at 25°C and then equilibrated in a dark incubator for an additional 22 h. At the end of the designated time, the suspensions were centrifuged at 10,000×g relative centrifugal force for 5 min and filtered through a 0.25-μm filter membrane. Total amounts of adsorbed iodine (I−, IO3−) were calculated by the difference between the total applied iodine and the iodine in the equilibrium solution by the following equation: Ia =(1-Ce/Co)VCo/g where Ia is the amount of iodine adsorbed (μg/g), V is volume of solution added (mL), Co is initial concentration of iodine (μg/mL), Ce is final equilibrium concentration of iodine (μg/mL), and g is weight of the soil taken.
- Desorption of Adsorbed Iodine:
The tubes (treated for the adsorption study) with the soil residue separated from the supernatant solution by centrifugation were weighed to measure the residual iodine in the soil. Then, 10 mL of 0.01 M CaCl2 solution was added to each tube containing the iodine-enriched soil residues. The suspensions were shaken at 160 rpm for 40 h at 25°C and equilibrated for an additional 22 h. The equilibrated suspensions were then centrifuged at 10,000×g relative centrifugal force for 5 min and filtered. The non-extractable fraction of the adsorbed iodine was obtained by the difference between the total adsorbed iodine and the total recovered iodine by extraction with the CaCl2 solution. - Computational methods:
- Langmuir equation: 1/y =1/ym + 1/(ymk1)(1/c)
Freundlich equation: y = k2c^1/n
Here, y is the amount adsorbed (mg/kg soil), ym is the maximum sorption value, c is the equilibrium concentration in solution (mg/L) and k1 and k2 are constants.
Distribution coefficient (Kd), which reflects the ratio of adsorbed iodine to dissolved iodine, was calculated as follows:
Kd (l/kg) = [iodine]s /[iodine]w
where [iodine]s is the uptake of iodine on soil particles from the solution and [iodine]w is the equilibrium concentration of iodine in the solution. - Conclusions:
- At the highest level of added iodine (200 mg/kg), the three soils (REQ, IS, and AS) adsorbed about 36.8%, 24.8%, and 15.9% of the applied iodate as compared with 17.7%, 13.1%, and 7.8% of the applied iodide, respectively.
The desorption of iodine in the soil increased with increasing adsorption. Between the two forms of iodine, the percentage of iodide desorbed after adsorption ranged from 12.6% to 35.3% of the total adsorption, while the corresponding value of iodate was from 8.1% to 24.7%.
At the same iodine loadings, the REQ has a greater affinity for iodine than the IS and AS. - Executive summary:
Adsorption–desorption of iodine in two forms, viz., iodide (I−) and iodate (IO3−), in three types of soil were investigated. The soils were: red soil developed on Quaternary red earths (REQ) -clayey, kaolintic thermic plinthite Aquult, Inceptisol soil (IS) and alluvial soil (AS)-Fluvio-marine yellow loamy soil. The isothermal curves of iodine adsorption on soils were described by Langmuir and Freundlich equation, and the maximum adsorption values (ym) were obtained from the simple Langmuir model.
At the highest level of added iodine (200 mg/kg), the three soils (REQ, IS, and AS) adsorbed about 36.8%, 24.8%, and 15.9% of the applied iodate as compared with 17.7%, 13.1%, and 7.8% of the applied iodide, respectively. Between the two forms of iodine, the percentage of iodide desorbed after adsorption ranged from 12.6% to 35.3% of the total adsorption, while the corresponding value of iodate was from 8.1% to 24.7%.
As compared with the iodide, the iodate was adsorbed in higher amounts by the soils tested. Among three soils, the REQ soil adsorbed more iodine (I- and IO3-) than the IS and AS. The distribution coefficient (Kd) of iodine in the soils decreased exponentially with increasing iodine loading concentration. Desorption of iodine in soil was increased correspondingly with increasing adsorption values. The REQ soil had a greater affinity for iodine than the IS and AS at the same iodine loadings.
- Endpoint:
- adsorption / desorption, other
- Remarks:
- adsorption
- Type of information:
- other: Handbook/compendia data
- Adequacy of study:
- supporting study
- Reliability:
- 2 (reliable with restrictions)
- Rationale for reliability incl. deficiencies:
- other: The presented data are per-reviewed by the scientific community and summarized from the literature by Sheppard et al. for modelling of soil transport for the assessment of the disposal concept of radioactive waste.
- GLP compliance:
- not specified
- Type of method:
- other: Data compilation
- Media:
- soil
- Type:
- Kd
- Value:
- > 0.04 - < 81 L/kg
- Remarks on result:
- other: sand soil (>= 70 % sand-sized particles)
- Type:
- Kd
- Value:
- > 0.1 - < 43 L/kg
- Remarks on result:
- other: loam soil (up to 80% silt-sized particles)
- Type:
- Kd
- Value:
- > 0.2 - < 29 L/kg
- Remarks on result:
- other: clay soil (>= 35% clay-sized particles)
- Type:
- Kd
- Value:
- > 1.4 - < 368 L/kg
- Remarks on result:
- other: organic soil (> 30% organic matter)
- Validity criteria fulfilled:
- not applicable
- Remarks:
- Data from handbook or collection of data
- Conclusions:
- The presented data from the compilation support the findings of Ashworth and Shaw.
The geometric mean Kd values for the different soil types are 1, 5, 1, 25 for sand, loam, clay and organic soil respectively. - Endpoint:
- adsorption / desorption: screening
- Type of information:
- read-across from supporting substance (structural analogue or surrogate)
- Adequacy of study:
- supporting study
- Reliability:
- 2 (reliable with restrictions)
- Rationale for reliability incl. deficiencies:
- study well documented, meets generally accepted scientific principles, acceptable for assessment
- Qualifier:
- no guideline followed
- Principles of method if other than guideline:
- The sorption of iodide by several soil component materials was examined over a range of pH values and compared with sorption by a surface soil.
- GLP compliance:
- not specified
- Media:
- soil
- Details on matrix:
- The soil was obtained from the 0 to 10 cm layer of an area of permanent pasture at Hurley, Berkshire. It had a pH of 6.7 (assessed in a 1:2.5 suspension with 0.01 M-CaCI2) and contained 3.70 % organic carbon (assessed by dichromate oxidation), 1.56% "free" ferric oxide and 0.24 % "free" aluminium oxide. The moist soil was stored in a refrigerator at 5℃.
- Details on test conditions:
- The solid:solution ratio was 1:100. Two iodide concentrations were used, 10 and 40 μg I/ 100 mL (7.88E-7 and 3.15 E-6 M) as KI.
Soil samples of 2.5 g were shaken with 250 mL of solution in glass tubes fitted with polyethylene caps, on an end-over-end shaker. In making the volume to 250 mL allowance was made for the water content of the samples and this resulted in slight variations in the concentration of calcium chloride used as the supporting electrolyte. Two drops of chloroform were added to suppress microbial activity. The samples were shaken for 40 hours at normal laboratory temperature (15 to 23 ℃): they were then centrifuged and, if necessary, filtered. The amounts of iodide sorbed were assessed by difference, from the concentrations of soluble inorganic iodine in the solutions shaken with the soil, compared with control solutions prepared without the addition of these materials. - Conclusions:
- The sorption of iodide by the soil was assessed at iodide concentrations of 10 and 40 μg I/ 100 mL and at two pH values, four replicates each. Substantial amounts of iodide were sorbed by the soil, which were 7.3, 27.5 μg I/g soil for initial concentration of 10 μg I/ 100 mL at pH 4.5 and 7.6, 10.0 μg I/g soil for initial concentration of 40 μg I/ 100 mL at pH 6.8. The sorption of iodide by surface soils decreased with pH increased during Ca. pH2.5-6.8, small increased in range during ca. pH 6.8-8 and then decreased again with pH increased (pH8-10). Drying markedly reduced the capacity for iodide sorption of soil.
- Executive summary:
The sorption of iodide by soil was examined over a range of pH values. The sorption of iodide by the soil was assessed at iodide concentrations of 10 and 40 μg I/ 100 mL and at two pH values, four replicates each. Substantial amounts of iodide were sorbed by the soil, which were 7.3, 27.5 μg I/g soil for initial concentration of 10 μg I/ 100 mL at pH 4.5 and 7.6, 10.0 μg I/g soil for initial concentration of 40 μg I/ 100 mL at pH 6.8. The sorption of iodide by surface soils decreased with pH increased during Ca. pH2.5-6.8, small increased in range during ca. pH 6.8-8 and then decreased again with pH increased (pH8-10). Drying markedly reduced the capacity for iodide sorption of soil.
Referenceopen allclose all
The conditions in the 25 % moisture content columns led to oxic condtions which were maintained over the whole period of 49 days. For the 40 % moisture content columns similarly conditions were predominant over the first 30 days before the redox potential fall to -100 mV by the end of the experiments. The analysis of the nitrate and sulphate concentrations showed no significantly decrease for the drier columns. In contrast, the nitrate concentration in the saturated clolumns decreased by about 60 %.
The Kd values were calculated on basis of the sampling results using the following equitation:
Kd= ((T ρ)-(θSn))/ρ/Sn
T: total soil concentration (soil+solution) [per kg soil]
ρ: soil bulk density [kg/L]
θ: soil volumetric moisture content [dim.less]
Sn: solution phase concentration [per L solution]
The concentration values were substituted by measured specific activity (Bq/kg or Bq/L). The 'total' activity of the soil was taken from the initial measurements before the columns were packed.
Over the first two weeks of the experiment the Kd values for both treatments (25% and 40% soil moisture) were below 1 L/kg. Afterwards the Kd values tended to increase with time with a higher increase for the drier columns (oxic conditions). By end of the experiment the Kd value for the drier (oxic) conditions stabilized at 7 L/kg, while the Kd value for the saturated (anoxic) conditions stabilized at 2 L/kg.
- Adsorption Isotherms of Iodine:
Iodine applied at 0~20 mg/kg was mostly adsorbed in the soils. Adsorption of iodine increased steeply with iodine addition in the equilibrium solution at low concentrations (0-2 mg/kg) for these soils, whereas this sharp increase started diminishing at the equilibrium iodine concentration >2 mg/kg. The REQ soil had the highest capacity to adsorb iodine, while the AS had the lowest uptake value.
The ym values were 30.4, 24.6, and 16.6 mg/kg for REQ, IS, and AS, respectively, if the soils were treated with iodide, and the corresponding values were 68.0, 43.7, and 33.7 mg/kg for iodate added to these soils. At the highest level of added iodine (200 mg/kg), the three soils (REQ, IS, and AS) adsorbed about 36.8%, 24.8%, and 15.9% of the applied iodate as compared with 17.7%, 13.1%, and 7.8% of the applied iodide, respectively. The affinity of iodine to REQ was at the highest among three soils. As compared with AS, the IS had higher iodine adsorption, indicating that the capacity of iodine adsorption was also related with pH, CEC, organic matter, and clay particles in soil.
There was variation in iodine adsorption on soils mainly favored by higher contents of Fe/Al oxide, clay and organic matter, and lower pH values.
- Desorption of Iodine
Iodine desorption was enhanced with the increased saturation of iodine adsorption in three soils. Between the two forms of iodine, the percentage of iodide desorbed after adsorption ranged from 12.6% to 35.3% of the total adsorption, while the corresponding value of iodate was from 8.1% to 24.7%.It indicated that iodide was adsorbed less and desorbed more. The amounts of iodine desorbed from soil also differed greatly among the soil types. The maximum amount of iodide and iodate desorbed accounted for 22.6% and 15.7% of the adsorbed for REQ, 27.2% and 19.4% for IS, and 35.3% and 24.7% for AS, respectively. Desorption percentage for REQ was significantly lower than in the other two soils, which confirmed that REQ had the highest adsorption capacity for iodine.
The amounts of iodine desorbed were positively correlated with those of iodine adsorption for the three soils.
- Distribution Coefficients of Iodine:
Kd values varied greatly from 0.85 to 69.6 among the three soils. Iodine was adsorbed almost completely at low concentrations (<10 mg/kg) with higher Kd values. Then, it decreased sharply with increasing initial iodine concentrations from 10 to 50 mg/kg and decreased slowly at further higher iodine concentrations (>100 mg/kg).
Between the two forms of iodine, the Kd of iodate was higher as compared to iodide, which indicated that the iodate was inclined to be adsorbed by solid partition. Among the three soils, REQ had a much higher Kd value than IS and AS.
The geometric mean Kd values for the different soil types are:
soil type | geometric mean [L/kg] |
sand soil | 1.0 |
loam soil | 5 |
clay soil | 1 |
organic soil | 25 |
- Experiment 1. Sorption of iodide by soil:
The sorption of iodide by the soil was assessed at iodide concentrations of 10 and 40 μg I/ 100 mL and at two pH values, four replicates each. Substantial amounts of iodide were sorbed by the soil, which were 7.3, 27.5 μg I/g soil for initial concentration of 10 μg I/ 100 mL at pH 4.5 and 7.6, 10.0 μg I/g soil for initial concentration of 40 μg I/ 100 mL at pH 6.8.
- Experiment 2. The influence of pH on the sorption of iodide by soil
In this experiment, the initial iodide concentration was 40 μg/100 mL and a range of pH values was obtained by including graded amounts of 0.1 N-HCI or NaOH in the 0.01 M-CaCI2 used as supporting electrolyte. The sorption of iodide by surface soils decreased with pH increased during Ca. pH2.5-6.8, small increased in range during ca. pH 6.8-8 and then decreased again with pH increased (pH8 -10).
- Experiment 3. The influence of drying on the sorption of iodide by soil
Samples of the four materials were subjected to (i) freeze drying for 24 h, (ii) drying at 27℃for 48 h, (iii) drying at 65℃for 24 h and (iv) drying at 100℃ for 24 h. Their sorption of iodide was then compared to sorption by undried samples at initial concentrations of iodide of 10 and 40 μg/100 mL. Sorption was assessed at pH 5.7 for soil. Drying decreased the capacity for iodide sorption particularly when it was carried out at elevated temperatures.
Description of key information
For determination of the Koc value the report of Ashworth is used. Based on the final result for oxic conditions a value of 7.7 L/kg is observed after 49 days. The organic partition is about 4.7 %. Thus, according to the TGD R.16 eq. R16-6 the Koc value is 1.64 L/kg. For anoxic conditions the Koc is 0.47 L/kg.
Key value for chemical safety assessment
- Koc at 20 °C:
- 1.64
Other adsorption coefficients
- Type:
- log Kp (solids-water in soil)
- Value in L/kg:
- 0.89
- at the temperature of:
- 20 °C
Additional information
Although the data were not obtained according a standard test guideline and GLP, the new mini-column approach of Ashworth et al. (Ashworth, 2006) can be supposed to be adequate and reliable for the environmental risk assessment of iodine.
In the study the long-term behavior of iodine under more realistic soil moisture conditions as well as the changes in behavior with time-dependent variations of the soil redox conditions is observed. In contrast to a short-term batch equilibrium HPLC method this long-term experiment gives the opportunity of a more exact examination of the partitioning of a chemical in soil. In addition, Ashworth et al. (Ashworth, 2006) were able to observe the behavior of iodine in oxic and anoxic soil redox conditions.
The obtained results for iodine seem to be consistent with geometric mean compendia values and indicate that iodine within oxic environments is less mobile and, probably, less bio-available than in anoxic environments.
Information on Registered Substances comes from registration dossiers which have been assigned a registration number. The assignment of a registration number does however not guarantee that the information in the dossier is correct or that the dossier is compliant with Regulation (EC) No 1907/2006 (the REACH Regulation). This information has not been reviewed or verified by the Agency or any other authority. The content is subject to change without prior notice.
Reproduction or further distribution of this information may be subject to copyright protection. Use of the information without obtaining the permission from the owner(s) of the respective information might violate the rights of the owner.
