Registration Dossier
Registration Dossier
Data platform availability banner - registered substances factsheets
Please be aware that this old REACH registration data factsheet is no longer maintained; it remains frozen as of 19th May 2023.
The new ECHA CHEM database has been released by ECHA, and it now contains all REACH registration data. There are more details on the transition of ECHA's published data to ECHA CHEM here.
Diss Factsheets
Use of this information is subject to copyright laws and may require the permission of the owner of the information, as described in the ECHA Legal Notice.
EC number: 230-386-8 | CAS number: 7085-19-0
- Life Cycle description
- Uses advised against
- Endpoint summary
- Appearance / physical state / colour
- Melting point / freezing point
- Boiling point
- Density
- Particle size distribution (Granulometry)
- Vapour pressure
- Partition coefficient
- Water solubility
- Solubility in organic solvents / fat solubility
- Surface tension
- Flash point
- Auto flammability
- Flammability
- Explosiveness
- Oxidising properties
- Oxidation reduction potential
- Stability in organic solvents and identity of relevant degradation products
- Storage stability and reactivity towards container material
- Stability: thermal, sunlight, metals
- pH
- Dissociation constant
- Viscosity
- Additional physico-chemical information
- Additional physico-chemical properties of nanomaterials
- Nanomaterial agglomeration / aggregation
- Nanomaterial crystalline phase
- Nanomaterial crystallite and grain size
- Nanomaterial aspect ratio / shape
- Nanomaterial specific surface area
- Nanomaterial Zeta potential
- Nanomaterial surface chemistry
- Nanomaterial dustiness
- Nanomaterial porosity
- Nanomaterial pour density
- Nanomaterial photocatalytic activity
- Nanomaterial radical formation potential
- Nanomaterial catalytic activity
- Endpoint summary
- Stability
- Biodegradation
- Bioaccumulation
- Transport and distribution
- Environmental data
- Additional information on environmental fate and behaviour
- Ecotoxicological Summary
- Aquatic toxicity
- Endpoint summary
- Short-term toxicity to fish
- Long-term toxicity to fish
- Short-term toxicity to aquatic invertebrates
- Long-term toxicity to aquatic invertebrates
- Toxicity to aquatic algae and cyanobacteria
- Toxicity to aquatic plants other than algae
- Toxicity to microorganisms
- Endocrine disrupter testing in aquatic vertebrates – in vivo
- Toxicity to other aquatic organisms
- Sediment toxicity
- Terrestrial toxicity
- Biological effects monitoring
- Biotransformation and kinetics
- Additional ecotoxological information
- Toxicological Summary
- Toxicokinetics, metabolism and distribution
- Acute Toxicity
- Irritation / corrosion
- Sensitisation
- Repeated dose toxicity
- Genetic toxicity
- Carcinogenicity
- Toxicity to reproduction
- Specific investigations
- Exposure related observations in humans
- Toxic effects on livestock and pets
- Additional toxicological data
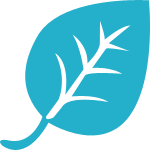
Phototransformation in water
Administrative data
Link to relevant study record(s)
- Endpoint:
- phototransformation in water
- Type of information:
- experimental study
- Adequacy of study:
- supporting study
- Study period:
- 27 October 1988 to 02 February 1990
- Reliability:
- 2 (reliable with restrictions)
- Rationale for reliability incl. deficiencies:
- guideline study with acceptable restrictions
- Study type:
- direct photolysis
- Qualifier:
- according to guideline
- Guideline:
- EPA Guideline Subdivision N 161-2 (Photodegradation Studies in Water)
- Deviations:
- no
- GLP compliance:
- yes
- Remarks:
- The work conducted after 16 October 1989, was conducted in accordance with the EPA GLP Standards.
- Specific details on test material used for the study:
- RADIOLABELLING INFORMATION
- Radiochemical purity: 96.5 % in solvent System A and 97.0 % in Solvent System B.
- Specific activity: 181 μCi/mg
- Locations of the label: U-ring-labelled. - Radiolabelling:
- yes
- Analytical method:
- liquid chromatography
- Details on sampling:
- Preliminary Investigation
Spectra-cells and glass vials were removed from the Chroma 50 artificial light source at the time intervals listed below and analysed as described in the analysis section. After analysis, the cells and vials were returned to the light source and used for the next sample interval.
Definitive Study
The photolysis and hydrolysis samples were analysed at the times listed, using procedures outlined in the analysis section. Sampling times were posted on a master calendar and were based on the results of the preliminary investigation, as well as previous analyses. After analysis, the photolysis samples were stored in a freezer. - Buffers:
- Solvent System A: Chloroform: hexane: glacial acetic acid (80:20:10)
Solvent System B: Toluene: methanol: glacial acetic acid (90:16:8)
Solvent System C: Acetonitrile: water: ammonium hydroxide (80:18:2)
pH 5 Buffer: 0.01 M sodium acetate adjusted to pH 4.99 and pH 5.00 with acetic acid for preliminary and definitive studies, respectively.
pH 7 Buffer: 0.01 M potassium phosphate adjusted to pH 7.00 and pH 7.00 with acetic acid for preliminary and definitive studies, respectively.
pH 9 Buffer: 0.01 M sodium borate adjusted to pH 9.00 and pH 9.01 with sodium hydroxide and/or acetic acid for preliminary and definitive studies, respectively. - Light source:
- other: Sunlight and artificial sunlight
- Relative light intensity:
- 320 - 3 500
- Details on light source:
- Chroma50 artificial light source.
The intensity of the artificial sunlight and natural sunlight was measured with a Blak-Ray UV meter.
The intensity of the Chroma 50 artificial light source was measured. At a distance of 9 cm, equal to the placement distance of the Spectra-cells, the intensity of the Chroma 50 lamps was 320 i.W/cm^2.
Natural sunlight was measured with the Blak-Ray meter at various times, dates, and conditions. - Type of sensitiser:
- not specified
- Details on sensitiser:
- Acetone
- Details on test conditions:
- TEST SYSTEM
- Type, material and volume of test apparatus/vessels: Aliquots (4.0 mL) of each aqueous buffer solution were placed in separate cells and vials and capped. All vials and cells were labelled with a unique sample number, the study number, and a description of the matrix. All glass vials were wrapped in aluminium foil to prevent light exposure.
- Sterilisation method: The cells and vials were sterilised by autoclaving for 4.25 hours at 122 °C.
TEST MEDIUM
- Preparation of test medium: The fortification solution was prepared by mixing 14C-test material and analytical-grade test material in ACN. Each vial and cell were fortified with 40 µL of the test material solution. Pre-, mid-, and post-fortification aliquots were removed from the test material solution to determine the amount of test material applied. The final concentrations in the cells and vials were 51 ppm (3.8 µCi) for all pH 9 samples; 52 ppm (4.4 µCi) for the pH 5 and 7 non-sensitised, and dark control; and 51 ppm (4.4 µCi) for the pH 5 and 7 sensitised samples.
- To one set of Spectro-cells at each pH, 40 µL of acetone was added as a sensitiser.
- The photolysis cells and vials were placed in an inverted position between four Chroma 50 artificial lamps (two on each side) and maintained at 25 ± 1°C in a gentle airflow stream. The temperature of the Chroma 50-exposed samples was monitored using a temperature probe inserted into an unfortified sample cell. Temperature was recorded using a Dickson Minicorder.
REPLICATION
- For each of the three pH groups there were two sets of glass vials (five vials each) and two sets of Spectro-cells (five cells each).
OTHER
Preliminary Investigation
- Sample Preparation and Fortification: Aliquots (3.5 mL) of each aqueous buffer solution were placed in separate glass vials (three) and Spectra-cells (six). These were capped and autoclaved for 4 hours at 122 °C. To minimise the headspace, an additional 0.5 mL of each buffer solution was added for a total of 4.0 mL. To three of the Spectra-cells, 40 µL of acetone was added as a sensitiser. The glass vials were wrapped with aluminium foil. The fortification solution was prepared by mixing 14C-test material and analytical-grade the test material in acetonitrile (ACN). Each vial and cell were fortified with 30 µL of the test material solution to obtain a final concentration of 41 ppm [1.2 µCi 14C-test material as determined by liquid scintillation counting (LSC)]. The cells and vials were placed in an inverted position between four Chroma 50 artificial lamps (two on each side) and maintained at approximately 25 °C. - Computational methods:
- The percent of the test material remaining unchanged was calculated by multiplying the percent recovery value by the percent of the test material as determined by the linear analyser. Sufficient decomposition did not occur in the dark control, thus its half-life was neither calculated nor subtracted from the photolysis data.
- Preliminary study:
- TLC of photolysed samples in Solvent System A separated two regions of radioactivity, one corresponding to the test material and the other to origin material. The 14C-content of the test material peak and of the origin were quantitated. The results indicated that rates of degradation were greatest in the pH 9 sensitised samples and declined in lower pHs and non-sensitised samples. Unexposed samples appeared to be stable during the test. Percent recovery ranged from 83.9 % to 105 % with a mean of 96.1 %. Declining recoveries occurred in the sensitised samples, thus indicating formation of volatile products. Validation of the TLC procedure provided 91 % to 94 % recovery. Final pH values remained within 0.14 pH of the initial pH values. The results of the preliminary investigation indicated the following precautions should be observed in the definitive study: The solvent system should be made regularly, the tanks should be saturated before development, and headspace should be reduced in the sample containers to minimise volatility.
- DT50:
- 680 h
- Test condition:
- pH 5
- DT50:
- 1 019 h
- Test condition:
- pH 7
- DT50:
- 415 h
- Test condition:
- pH 9
- Details on results:
- Individual recoveries ranged from 77.5 % to 117.0 %. The mean of all values was 105 %. Low recoveries observed from the first time interval of pH 9 samples were attributed to inadequate mixing, and these values were excluded. Investigation of the anomalous recoveries from the pH 5, 748-hour samples revealed that the sample had not been fortified. Recoveries dropped in the sensitised matrices of pH 5 and 7 to values of 77.5 % and 79.5 %, respectively. The loss of activity is considered to be due to formation of volatile products. Investigation of the anomalous recoveries from the pH 5, 748-hour samples revealed that the sample had not been fortified. Recoveries dropped in the sensitised matrices of pH 5 and 7 to values of 77.5 % and 79.5 %, respectively. The loss of activity is considered to be due to formation of volatile products.
Final pH values remained within 0.57 pH from the initial values. Recoveries for the TLC procedure validation ranged from 97.6 % to 100.4 %.
Using Solvent System A, the test material was separated and quantified, and these values were used in the estimated half-life calculation. The calculated half-life of the test material in sensitised aqueous buffer was 72, 70, and 44 hours at pH 5, 7, and 9, respectively. In non-sensitised aqueous buffer, the estimated half-life was 1 000, 1 060, and 770 hours in pH 5, 7, and 9, respectively. The origin material present in Solvent System A was separated using Solvent System C. This resolved two major radioactive areas and one minor radioactive area in addition to origin material and the test material. - Validity criteria fulfilled:
- not specified
- Conclusions:
- Under the conditions of the study, degradation of the test material in aqueous buffer solutions was photolytically driven. The half-life was calculated to be 72, 70, and 44 hours in sensitised aqueous buffer at pH 5, 7, and 9, respectively. In non-sensitised aqueous buffer, the calculated half-life was 1 000, 1 060, and 770 hours at pH 5, 7, and 9, respectively. Two major photoproducts (greater than 10 % of applied radioactivity) and several minor products were formed in some of the sensitised and non-sensitised samples. Because of the further separation of radioactive components in this investigation, the degradation half-lives of 14C-test material were recalculated to be: 680 (pH 5), 1 019 (pH 7), and 415 (pH 9) hours.
- Executive summary:
The phototransformation in water of the test substance was assessed according to EPA Guideline Subdivision N 161-2 and according to the principles of GLP.
Spectro-cells and glass vials that contained sterile solutions of test material buffered at pH 5, 7, and 9 were prepared. One set of cells was fortified with acetone to sensitise the solution, and another set of cells remained non-sensitised. One set of glass vials was wrapped in aluminium foil and placed with the cells for dark controls. The cells and vials were exposed to Chroma 50® artificial sunlight. They constituted the photolysis portion of the study. At various times cells and vials were removed and analysed for evidence of degradation of the test material. Photolytic degradation of the test material occurred in both the sensitised and non-sensitised aqueous buffer solutions.
Under the conditions of the study, degradation of the test material in aqueous buffer solutions was photolytically driven. The half-life was calculated to be 72, 70, and 44 hours in sensitised aqueous buffer at pH 5, 7, and 9, respectively. In non-sensitised aqueous buffer, the calculated half-life was 1 000, 1 060, and 770 hours at pH 5, 7, and 9, respectively. Two major photoproducts (greater than 10 % of applied radioactivity) and several minor products were formed in some of the sensitised and non-sensitised samples. Because of the further separation of radioactive components in this investigation, the degradation half-lives of 14C-test material were recalculated to be: 680 (pH 5), 1 019 (pH 7), and 415 (pH 9) hours.
- Endpoint:
- phototransformation in water
- Type of information:
- experimental study
- Adequacy of study:
- key study
- Study period:
- 07 April 1999 to 02 June 1999
- Reliability:
- 1 (reliable without restriction)
- Rationale for reliability incl. deficiencies:
- guideline study
- Qualifier:
- according to guideline
- Guideline:
- other: Part I, Section 10 of Procedures for Assessing the Environmental Fate and Ecotoxicity of Pesticides published by SET AC-Europe, 1995.
- Deviations:
- no
- Qualifier:
- according to guideline
- Guideline:
- other: Section 7.2.1.2 of Annex II of Directive 91/414/EEC
- Deviations:
- no
- Qualifier:
- according to guideline
- Guideline:
- other: JMAFF Guidelines
- Deviations:
- no
- GLP compliance:
- yes
- Analytical method:
- high-performance liquid chromatography
- Light source:
- Xenon lamp
- Light spectrum: wavelength in nm:
- > 290
- Relative light intensity:
- 425
- Details on light source:
- The test solutions for exposure were irradiated in Heraeus Suntest CPS equipment fitted with a xenon arc light source (which emits a spectrum similar to that of natural sunlight) and filters to cut-off light of less than 290 nm wavelength (Heraeus Equipment Limited, Brentwood, Essex). The intensity was set at 425 W m^-2 and monitored using a Radialux UV measuring instrument (Heraeus Equipment Limited, Brentwood, Essex).
The photolysis cells were 50 mL capacity glass containers, with an outer glass jacket for circulating water and screw cap fitted with a quartz top window. The temperature was monitored using a Portee PI 8013 type K thermocouple thermometer (Portee Instrumentation Ltd, Milton Keynes, Bucks). - Details on test conditions:
- TEST MEDIUM
- Source of natural water: The natural water used in this study was sampled from the middle pond of "Five Row Ponds'', a perennial pond system fed by spring water and drainage off parkland and Hardwick Hall roof. This water is associated with a silty clay sediment, and was passed through a 0.2 mm sieve prior to use in this study. Following sampling and receipt at Huntingdon Life Sciences, the water was stored at approximately 4 °C in the dark until it was used in the study. The characteristics of the water were as follows:
Location: Row Pond, Hardwick Hall, Derbyshire
Total organic carbon (ppm): 3.8
Total nitrogen (ppm): 2.0
Total phosphorus (ppm): 0.2
Total hardness ((ppm) CaC03 equiv.): 229
pH at sampling: 7.61
Conductivity (µSiem): 474
Oxygen content (%) at below surface: 86
Sampling 5 cm above sediment: 75
Redox potential (m V) at sampling: 4
- Preparation of test medium: Test solution concentration and preparation of fortification solutions: The test solution concentration is required to be less than half the water solubility, which for the test material is 734 mg/L at 25 °C. In addition, the concentration should be such that its absorbance is less than 0.05 absorbance units (AU) at wavelengths greater than 290 nm (U.S. E.P.A. document CG-6000, "Photolysis in Aqueous Solution in Sunlight", October 1983). Ultraviolet light spectra for the test material in water were obtained over the range 290 to 800 nm. At a concentration of 9.7 mg/L the test material showed a wavelength maximum at 290 nm of 0.032 AU. Thus, the nominal test solution concentration selected for the study was 10 mg/L. Fortification solutions were prepared at a concentration of 2.5 mg/mL by dissolving the test material in acetonitrile.
- Fortification of test solutions: Portions of distilled water (50 mL) or natural water (250 mL) were transferred to respective test vessels (photolysis cells for light irradiated samples, and Wheaton vials or glass bottles for dark control samples). Aliquots of the fortification solution (200 µL or 1 mL) were transferred to each of the test vessels to produce a nominal test material concentration of 10 mg/L, with an acetonitrile content of 0.4 % by volume. At the time of treatment aliquots of the fortification solution, of the same volume as that required to achieve the nominal application rate, were taken and diluted to known volumes (50 or 250 mL) with distilled water. Aliquots were taken from each diluted sample for HPLC/UV analysis.
REPLICATION
- No. of replicates (dark): Duplicate dark control samples.
OTHER
- Preparation of sterilised distilled water test systems: The distilled water and all glassware and associated equipment required for the sterile test were autoclaved at 121 °C and 15 lb/in^2 for 15 minutes prior to use. All procedures involving preparation of the test systems (for example, dispensing volumes of sterile distilled water into glassware and fortification of test systems) were conducted in a laminar flow cabinet to ensure maintenance of sterility.
- The photolysis vessels were connected to a circulating water system inside the Heraeus Suntest CPS exposure unit. Water was circulated continuously throughout the test to maintain the temperature at approximately 25 °C. The temperature was monitored daily using a thermocouple inserted directly into a monitoring vessel (set up exactly as the test vessels) and connected to a thermocouple thermometer to provide a digital readout.
The xenon arc lamp was ignited and the intensity adjusted to 425 W m^2 by measuring the irradiance with the Heraeus Radialux exposure monitoring device and global sensor. The samples were irradiated continuously, unexpected interruptions to the light supply being corrected by extending the irradiation period. Irradiation time was measured in hours of continuous exposure.
For the definitive test, duplicate dark control samples were prepared for each treatment and were incubated in the dark in an incubation room, at a target temperature of 25 ± 2 °C. The temperature was monitored with a minimum/maximum thermometer over the test period to ensure the temperature was maintained.
The study comprised a preliminary test with non-sterile distilled water (bulk sample from which sub-samples were removed for analysis at each timepoint), from which the data was used to select sampling points for the definitive tests conducted with sterile distilled water (individual 50 mL samples which were removed for analysis at each timepoint) and natural water (bulk sample from which sub-samples were removed for analysis at each timepoint). General conditions for each of the tests were as follows:
- Preliminary Test (non-sterile distilled water): Light irradiated 0, 16.5 and 112.4 h. Triplicate sub-samples were removed from the test vessel for HPLC/UV analysis.
- Definitive Test (sterile distilled water): Light irradiated 0, 6, 24, 51, 72, 99 and 120 h.
Dark controls 0, 48 and 120.5 h.
Duplicate test vessels were removed and aliquots (approximately 1.5 mL) taken from each for HPLC/UV analysis.
- Definitive Test (natural water): Light irradiated: 0, 6, 24, 48, 72, 100, 123, 170 and 219 hours.
Dark control: 0, 6, 24, 48, 72, 100.5, 124, 170.5 and 220 hours.
Sub-samples (approximately 5 mL) were removed from duplicate test vessels for HPLC/UV analysis.
- Sterility analysis: The aliquots taken for sterility analysis at each sampling occasion during the definitive test were despatched to the Department of Microbiology, Huntingdon Research Centre, Cambridgeshire. Duplicate aliquots (1 mL) were applied to molten tryptone soya agar pour plates and incubated at 30 – 35 °C for not less than 5 days. After incubation, if any colonies were present they would be counted and the level of contamination calculated per mL of sample reported. - Duration:
- 120 h
- Dark controls:
- yes
- Key result
- DT50:
- 76.2 h
- Test condition:
- Natural water
- Transformation products:
- not measured
- Details on results:
- APPLICATION RATE
- Ultraviolet/visible spectrum of the test material: An ultraviolet/visible absorption spectrum was obtained for the test material in distilled water. This gave an absorption maximum (λmax) at 290 nm. For a solution of 9.7 mg/L the absorbance at 290 nm was 0.032 AU. Therefore 10 mg/L was selected as the nominal application rate.
- HPLC analytical method: The analytical method was found to be linear over the range 0.5 to 50 mg/L. The limit of quantitation was not determined but was below 0.5 mg/L.
- Achieved application rate: The test systems were fortified with the test material to achieve a nominal concentration of 10 mg/L. The achieved application rates were between 97.4 % and 104.7 % of the nominal application rate.
- Test system monitoring during preliminary and definitive tests: The sterile distilled water test systems were shown to remain sterile throughout irradiation/incubation.
The temperature of the test systems, measured during light irradiation or incubation in the dark, was in the range 25 ± 2 °C throughout the study except on one occasion where the temperature increased to 32.5 °C. The temperature increased to 32.5 °C over a period of 40 minutes following the 51-hour sampling occasion during the sterilised distilled water test. Only the 72-hour samples were subjected to the higher temperature and therefore the outcome of the study was not affected.
The light intensity measurements taken during the preliminary and definitive tests were in the range 408 to 458 W m^-2.
PHOTODEGRADATION
- Preliminary test: The concentration of the test material in distilled water solution decreased from 10.47 mg/L at T0 to 0.43 mg/L (2.0 % of applied) at 112.4 hours.
- Sterile distilled water test: The test material was shown to degrade in sterile distilled water irradiated with a source of artificial sunlight. The concentration of the test material in irradiated solutions decreased from 10.06 mg/L at T0 to 0.89 mg/L at 120 hours. The test material was shown to be hydrolytically stable as no degradation was detected in the dark control samples. The concentration of the test material in the dark controls was 9.97 mg/L at 120 hours. The rate of photolytic degradation (Kp) of the test material was determined to be 0.0169 hours^-1 and the half-life to be 41.0 hours.
- Natural water test: The test material was shown to degrade in natural water irradiated with a source of artificial sunlight. The concentration of the test material in irradiated solutions decreased from 10.18 mg/L at T0 to 1.39 mg/L at 219 hours. The test material was stable in the dark controls as no biotic degradation was observed. The concentration of the test material in the dark controls was 10.14 mg/L at 220 hours. The rate of photolytic degradation (Kp) of the test material was determined to be 0.0091 hours^-I and the half-life to be 76.2 hours. - Validity criteria fulfilled:
- not specified
- Conclusions:
- Under the conditions of the test, the photodegradation of the test material in natural water was shown to follow first-order kinetic behaviour, and the derived photolysis rate constant (Kp) was determined to be 0.0091 hours^-1 and the half-life to be 76.2 hours
- Executive summary:
The photolysis rate constant and half-life of the test material in sterile distilled and natural water at 25 °C were assessed in order to meet the requirements of JMAFF Guidelines and Section 7.2.1.2 of Annex II of Directive 91/414/EEC.
The ultraviolet/visible absorption spectrum of the test material showed a wavelength maximum (λmax) at 290 nm. The absorbance at 290 nm was 0.032 AU for a solution of 9.7 mg/L. On this basis the test solution concentration for the test material (less than half-saturation and an absorbance of less than 0.05 AU at wavelengths greater than 290 nm) was selected to be 10 mg/L.
A preliminary photodegradation test was performed at 25 °C, by exposing cells containing the test material in distilled water to artificial sunlight (provided by a xenon arc lamp set at 425 W m^-2 inside the Heraeus Suntest CPS exposure unit). The test material was shown to degrade such that 2.0 % of the applied test material remained in solution after 112.4 hours.
On the basis of the results from the preliminary test, the photodegradation of the test material in sterile distilled water test was conducted with samples taken at T0, 6, 24, 51, 72, 99 and 120 hours. Hydrolytic stability of the test material was shown by the stability of the test material in dark controls. This allowed direct determination of the rate of photodegradation of the test material in solution. Photodegradation of the test material in sterile distilled water was shown to follow first-order kinetic behaviour, and the derived photolysis rate constant (Kp) was determined to be 0.0169 hours^-1 and the half-life to be 41.0 hours at 25 °C.
The photodegradation of the test material in natural water test was conducted with samples taken at T0, 6, 24, 48, 72, 100, 123, 170 and 219 hours. Biotic stability of the test material was shown by the absence of degradation of the test material in the dark controls. This allowed direct determination of the rate of photodegradation of the test material in solution.
Under the conditions of the test, the photodegradation of the test material in natural water was shown to follow first-order kinetic behaviour, and the derived photolysis rate constant (Kp) was determined to be 0.0091 hours^-1 and the half-life to be 76.2 hours.
- Endpoint:
- phototransformation in water
- Type of information:
- experimental study
- Adequacy of study:
- supporting study
- Study period:
- Not reported
- Reliability:
- 2 (reliable with restrictions)
- Rationale for reliability incl. deficiencies:
- study well documented, meets generally accepted scientific principles, acceptable for assessment
- Study type:
- direct photolysis
- Qualifier:
- no guideline followed
- Principles of method if other than guideline:
- The test material was irradiated under various conditions of pH, oxygenation and wavelengths in order to study the reactions involved in the aqueous phototransformation.
- GLP compliance:
- not specified
- Specific details on test material used for the study:
- - Purity: 96 %
The commercial product, which was slightly coloured, was purified by recrystallisation from water + ethanol (1 + 1 by volume) and obtained as colourless needles. Its purity was confirmed by NMR and high performance liquid chromatography (HPLC) analyses. - Radiolabelling:
- no
- Analytical method:
- gas chromatography
- high-performance liquid chromatography
- mass spectrometry
- other: UV-VIS and fluorescence spectrophotometry, NMR
- Buffers:
- To compare the photochemical behaviour of both forms of test material, solutions were irradiated either at pH 2.15 (in the presence of hydrochloric acid) or at pH 5.5 (phosphate buffer).
- Light source:
- other: Xenon lamp, Mercury lamp, Fluorescent lamps, Lamps (Philips HPW 125), Natural sunlight
- Details on light source:
- Several devices were used for the irradiation of solutions. For the determination of quantum yields, solutions were irradiated at 280 nm with a xenon lamp (1 600 W) equipped with a Schoeffel monochromator providing a parallel beam. Band width at mid-height was about 10 nm. The photon flow was evaluated at 3.7 x 10^14 photons/cm^2/s with potassium ferrioxalate.
Solutions were irradiated at 254 nm in a device consisting of a cylindrical mirror with an elliptic base, a low-pressure mercury lamp ('Germicide Lamp') located along one of the focal axes and a quartz cylindrical reactor (2 cm ID) located along the other focal axis. The average photon flow received in 30 mL was evaluated as 2.1 x 10^17 photons/s, using uranyl oxalate as the chemical actinometer. In order to isolate photoproducts, a solution (100 mL) was irradiated with six low pressure mercury lamps surrounded by a cylindrical mirror. The photon flow in 100 mL was evaluated at 2.8 x 10^18 photons/s with uranyl oxalate.
Two other devices were used to study the influence of irradiation wavelength. One consisted of six fluorescent lamps (Duke GL 20W) emitting at wavelengths longer than 275 nm with a maximum near 310 nm. Mercury lines at 365, 405 and 436 nm were also emitted, but were not involved in the direct phototransformation of the test material. The quartz reactor was placed along the symmetry axis. The photon flow received in 21.5 mL was evaluated at 9.9 x 10^16 photons/s; this value is lower than the total photon flow emitted by the lamp, since the actinometer absorbs only a low percentage of the energy emitted on lines 405 nm and 436 nm, but slightly higher than the photon flow really absorbed by the solution of test material.
In the other device, solutions were irradiated with lamps (Philips HPW 125) and maintained at room temperature with a cooling jacket. These lamps were medium pressure mercury lamps fitted with a black bulb in order to select the line at 365 nm. About 85, 6 and 2 % of the light was emitted at 365 nm, 334 nm and 313 nm respectively. With this device the photon flow was evaluated at 2.4 x 10^17 photons/s in 20 mL using the chemical actinometer Aberchrome 540. Potassium ferrioxalate is too sensitive in these conditions and uranyl oxalate is not sufficiently absorbing at 365 nm.
Solutions were also exposed in natural sunlight in a 'Pyrex' vessel. Experiments were carried out in Clermont-Ferrand (latitude 46 ° N, altitude 420 m) in September.
- Details on test conditions:
- - Deoxygenation
In order to study the influence of oxygen, solutions were deoxygenated by argon bubbling for 50 min. The reactor was closed with a septum and samples were collected with a syringe at different stages of the photochemical transformation. - Reference substance:
- not specified
- Dark controls:
- yes
- Computational methods:
- Not reported
- Transformation products:
- yes
- No.:
- #1
- No.:
- #2
- No.:
- #3
- No.:
- #4
- Details on results:
- - UV absorption
Molecular and anionic forms of the test material have similar UV spectra. They absorb at wavelengths shorter than 310 nm. The maximum of absorption of both forms is located at 280 nm, but the molar absorption coefficient is slightly higher for the anionic form (ε = 1 604 M^-1cm^-1) than for the molecular form (ε = 1 390 M^-1 cm^-1) . The absorption spectrum of the test material overlaps with that of sunlight in summer, since the wavelength of sunlight starts at about 295 nm at sea level. The overlap is smaller, but not negligible, in winter. It is shown experimentally that the test material may be phototransformed in September. However, in environmental conditions some induced photoreactions may also be involved.
- Irradiation of the anionic form
A solution 4.94 x 10^-4 M of test material buffered at pH 5.5 was irradiated at 254 nm in the presence and in the absence of oxygen. Similar UV spectra were observed in both cases. The absorption increases and a weak absorption band appears between 300 and 400 nm. In both cases two photoproducts appear on the HPLC chromatogram.
The same photoproducts are formed when solutions are irradiated between 275 and 350 nm in the presence or in the absence of oxygen. Thus the formation of products is affected neither by oxygen nor by the irradiation wavelength between 254 nm and 350 nm. (Absorption is very low at λ > 310 nm).
2-(4-hydroxy-2-methylphenoxy)propionic acid is clearly the main primary photoproduct. Its UV spectrum, obtained on a photodiode array detector, is similar to the spectrum of the test material. It was isolated by preparative HPLC and identified by MS and 1H NMR.
- Induced phototransformation
Fe(III) salts absorb in the range 300 - 400 nm and may induce the photooxidation of organic substrates present in the solution. Several processes may be involved, depending on the substrate. In the present work, Fe(III) perchlorate (10^-3 M) was used to induce the degradation of the test material in acidic solution (5.03 x 10^-4 M; pH 2.15) irradiated at 365 nm. The acidification was necessary to prevent the precipitation of Fe(III) hydroxide. After 30 min about 83 % of test material was transformed, whereas direct photolysis is negligible under these conditions. The main product is 4-chloro-o-cresol that accumulates in the solution.
Methanol was used as a quencher of ·OH to identify the role of these radicals in the reaction. In the presence of methanol (20 mL/L, about 0.49 M) transformation is inhibited by about 80 %, whereas about 99 % of ·OH is quenched. (Evaluated from the rate constant 9.7 x 10^8 M^-1 s^-1 for the reaction ·OH + CH3OH). It was deduced that hydroxyl radicals contribute to the formation of 4-chloro-o-cresol, but probably another pathway is also involved.
Nitrite and nitrate ions are also photochemical sources of hydroxyl radicals.
The quantum yield of photolysis is significantly higher with HNO2 (about 0.45) than with NO2- (0.015 - 0.07 according to the wavelength) and NO3- (0.009 - 0.017). Nitrite ions were preferred to nitrate ions in the present work since they absorb at longer wavelengths (maximum at 352 nm) than nitrate ions (maximum at 302 nm) and it is possible to excite them selectively at 365 nm in the presence of test material. A further advantage is that nitrite ions have a higher molar absorption coefficient and higher quantum yield, but unfortunately NO2- ions are good ·OH quenchers and the production of ·OH in a solution is only efficient at low concentrations of nitrite.
A 5.0 x 10^-4 M solution of test material with 5 x 10^-4 M sodium nitrite and acidified at pH 2.15 was irradiated at 365 nm. Several photoproducts were formed but only 4-chloro-o-cresol accumulated in the solution. The efficiency increased with increasing irradiation time and it may be assumed that 4-chloro-o-cresol is not only formed as a primary photoproduct. By comparison with a solution kept in the dark it was established that this transformation did not result from a thermic reaction of HNO2. The rate of formation of 4-chloro-o-cresol was significantly higher at pH 2.15 than at pH 5.15, due to the fact that the photolysis of HNO2 is more efficient than the photolysis of NO2-. - Validity criteria fulfilled:
- not applicable
- Conclusions:
- The formation of the main primary photoproducts resulting from the phototransformation of the test material is not influenced either by oxygen or by wavelength in the range 254 - 310 nm, but depends on pH (different photochemical behaviour of molecular and anionic forms): Heterolytic photohydrolysis is the main reaction observed with the anionic form, whereas a photochemical rearrangement is the main pathway with the molecular form. A number of other photoproducts were identified: o-cresol, 4-chloro-o-cresol and quinonic derivatives. The photoreactivity of the molecular form is interesting for comparison with other chloroaromatic pesticides, but generally it plays a minor role under environmental conditions.
Different photochemical reactivity was observed when solutions were irradiated in sunlight or nearUV light. Under these conditions 4-chloro-o-cresol is formed as the main photoproduct. This phenomenon confirms the wavelength effect previously reported in the case of 4-chloro-2-methylphenoxyacetic acid. It is attributed to reactions induced by quinonic compounds formed as intermediates.
The transformation of the test material can be induced by Fe(III) salts and nitrite ions, leading to the formation of 4-chloro-2-methylphenol as the main product. An oxidation of the acetic moiety by hydroxyl radicals may be suggested.
The test material does not accumulate in environmental conditions and induced reactions play a major role in the phototransformation. - Executive summary:
The test material was irradiated under various conditions of pH, oxygenation and wavelengths in order to study the reactions involved in the phototransformation. Four main photoproducts were identified: 2-(4-hydroxy-2-methylphenoxy)propionic acid, o-cresol, 2-(5-chloro-2-hydroxy-3- methylphenyl)propionic acid, and 4-chloro-o-cresol. When the anionic form of the test material was irradiated between 254 nm and 310 nm (UV-C or UV-B), 2-(4-hydroxy-2-methylphenoxy)propionic acid was the main photoproduct. At 254 nm its formation initially accounted for more than 80 % of the transformation. The reaction results from a heterolytic photohydrolysis. O-cresol accounted for only a low percentage of the transformation. The stoichiometry was different with the molecular form: The main photoproduct, 2-(5-chloro-2-hydroxy-3- methylphenyl)propionic acid, resulted from a rearrangement after a homolytic scission. Products 2-(4-hydroxy-2-methylphenoxy)propionic acid, o-cresol and 4-chloro-o-cresol were also formed as minor photoproducts. Some other minor photoproducts were also identified. In contrast, 4-chloro-o-cresol was the main photoproduct under sunlight irradiation or when solutions were irradiated in near-UV light (UV-A). This wavelength effect is attributed to the involvement of an induced phototransformation; 4-chloro-o-cresol is also the main photoproduct when the phototransformation is induced by Fe(III) perchlorate or nitrite ions. In usual environmental conditions the excitation of the molecular form is negligible and the phototransformation is mainly due to induced photoreactions.
- Endpoint:
- phototransformation in water
- Type of information:
- experimental study
- Adequacy of study:
- supporting study
- Reliability:
- 2 (reliable with restrictions)
- Rationale for reliability incl. deficiencies:
- study well documented, meets generally accepted scientific principles, acceptable for assessment
- Study type:
- direct photolysis
- Qualifier:
- no guideline followed
- Principles of method if other than guideline:
- The present study focuses on the direct phototransformation of aromatic derivatives. Its aim is to classify the main reactions into different groups and to examine the influence of the nature and position of substituents on the orientation of the reaction in order to get insight into and to bring out some rules for the chemical behaviour of common herbicides. Where relevant the influence of pH and irradiation wavelength is pointed out.
- GLP compliance:
- not specified
- Remarks:
- Publication
- Radiolabelling:
- no
- Analytical method:
- high-performance liquid chromatography
- Light source:
- other: Low pressure mercury lamps, fluorescent lamps or sunlight.
- Light spectrum: wavelength in nm:
- >= 290 - <= 365
- Details on light source:
- Solutions were irradiated at 254 nm using low pressure mercury lamps, between 290 and 350 nm with fluorescent lamps “Duke Sunlamp GL20”, at 365 nm with lamps “Mazda” MAW 125W-T or in sunlight. Lamps MAW 125W-T consist of a medium pressure mercury lamp surrounded by a filtering glass transmitting mainly the line at 365 nm. However, a low percentage of light is emitted at 313 and 334 nm.
- Reference substance:
- no
- Dark controls:
- no
- Transformation products:
- yes
- No.:
- #2
- No.:
- #4
- Details on results:
- Reactions observed in the phototransformation of aromatic pesticides can be divided into three main reactions:
- Reactions involving carbon-halogen bonds (photohydrolysis, reactions resulting from the intermediate formation of a carbene, photoreduction of carbon-halogen bond);
- Reactions concerning the aromatic ring (photorearrangement, photooxidation, photocyclisation);
- Reactions involving the aliphatic moiety (demethoxylation of methoxylated phenylureas, ether bond scission).
Photorearrangement is reported with many aromatic herbicides including the test material. It is generally admitted that the reaction involves a radical mechanism, the aliphatic radical released reacting on the positions of highest spin density i.e. in ortho and para positions with respect to OH or NH2 group. Only one isomer is formed when isoproturon is irradiated at 254 nm since para position is substituted, but no rearrangement is observed when a solution is irradiated at λ ≥ 275 nm. This wavelength effect is probably due to the fact that photorearrangement results from the excitation in a ππ∗ band as it was suggested by Shizuka and Tanaka.
Ether bond scission is reported with various herbicides and leads to the formation of corresponding phenols. This reaction may result from two distinct processes: Homolysis of the excited molecule and induced photooxidation. The formation of rearrangement products provides evidence for the occurrence of direct homolysis. The formation of the same products by irradiation of solutions of the test material containing TiO2, nitrite ions, ferric salts or quinonic derivatives at wavelengths where direct photolysis is not possible gives support for the occurrence of induced photooxydation. The involvement of these two pathways can explain the observed wavelength effect.
The test material is an example of complex mechanism. In some cases the irradiation of a pesticide leads to the formation of one major product by one of the reactions described above. Most often the photodegradation of a pesticide involves several pathways, in proportions depending on the conditions of irradiation. For example the photochemical behaviour of the test material, a systemic herbicide, depends on both pH and irradiation wavelength.
The pKa of the test material is 3.78. When the anionic form (at pH = 5.5) is irradiated at λ ≤ 350 nm photohydrolysis (formation of I) accounts for about 90 % of the transformation. The formation of 2-methylphenol (II) is also reported, but it is a minor pathway. These photoproducts also appear when an aqueous solution is irradiated at pH 2.15 in order to excite the molecular form, but they only account for a low percentage of the transformation. The main product (III) results from a photorearrangement, but some other products are detected, namely 4-chloro-2-methylphenol (IV) resulting from ether bond scission and the substituted quinone (V). In contrast, when an unbuffered solution of mecoprop is irradiated in sunlight or in near UV light (lamps with a main line at 365 nm and minor emission at 313 and 334 nm), the phototransformation is very slow and the main photoproduct is IV. With the molecular form, disappearance of the test material and formation of IV are reported to be self-accelerated. IV is also the main product when oxidation of the test material is photoinduced by TiO2, nitrite ions, ferric salts or methylbenzoquinone. From these observations it is deduced that formation of IV in sunlight is mainly due to oxidation photoinduced by quinonic derivatives such as product V and methylbenzoquinone.
Many reactions may occur in the phototransformation of aromatic pesticides. The orientation of the reaction depends on the nature of the aliphatic moiety and on the nature and position of substituents on the ring. The orientation of the reaction may also depend on pH (with ionisable molecules) and irradiation wavelength. The latter phenomenon may be due to the larger part taken by induced phototransformations at longer wave lengths. Consequently it is useful to study photochemical reactions in well defined laboratory conditions, but it is necessary to control that these reactions do really occur in environmental conditions. The irradiation of pesticides may increase the toxicity of solutions and it is useful to control that photoproducts do not accumulate in water. - Validity criteria fulfilled:
- not applicable
- Conclusions:
- The photochemical behaviour of the test material depends on both pH and irradiation wavelength.
The pKa of the test material is 3.78. When the anionic form (at pH 5.5) is irradiated at λ ≤ 350 nm photohydrolysis (formation of I) accounts for about 90 % of the transformation. The formation of 2-methylphenol (II) is also reported, but it is a minor pathway. These photoproducts also appear when an aqueous solution is irradiated at pH 2.15 in order to excite the molecular form, but they only account for a low percentage of the transformation. The main product (III) results from a photorearrangement, but some other products are detected, namely 4-chloro-2-methylphenol (IV) resulting from ether bond scission and the substituted quinone (V). In contrast, when an unbuffered solution of the test material is irradiated in sunlight or in near UV light (lamps with a main line at 365 nm and minor emission at 313 and 334 nm), the phototransformation is very slow and the main photoproduct is IV. With the molecular form, disappearance of the test material and formation of IV are reported to be self-accelerated. IV is also the main product when oxidation of the test material is photoinduced by TiO2, nitrite ions, ferric salts or methylbenzoquinone. From these observations it is deduced that formation of IV in sunlight is mainly due to oxidation photoinduced by quinonic derivatives such as product V and methylbenzoquinone. - Executive summary:
The study investigated the direct phototransformation of aromatic derivatives.
The photochemical behaviour of the test material depends on both pH and irradiation wavelength.
The pKa of the test material is 3.78. When the anionic form (at pH 5.5) is irradiated at λ ≤ 350 nm photohydrolysis (formation of I) accounts for about 90 % of the transformation. The formation of 2-methylphenol (II) is also reported, but it is a minor pathway. These photoproducts also appear when an aqueous solution is irradiated at pH 2.15 in order to excite the molecular form, but they only account for a low percentage of the transformation. The main product (III) results from a photorearrangement, but some other products are detected, namely 4-chloro-2-methylphenol (IV) resulting from ether bond scission and the substituted quinone (V). In contrast, when an unbuffered solution of the test material is irradiated in sunlight or in near UV light (lamps with a main line at 365 nm and minor emission at 313 and 334 nm), the phototransformation is very slow and the main photoproduct is IV. With the molecular form, disappearance of the test material and formation of IV are reported to be self-accelerated. IV is also the main product when oxidation of the test material is photoinduced by TiO2, nitrite ions, ferric salts or methylbenzoquinone. From these observations it is deduced that formation of IV in sunlight is mainly due to oxidation photoinduced by quinonic derivatives such as product V and methylbenzoquinone.
- Endpoint:
- phototransformation in water
- Type of information:
- experimental study
- Adequacy of study:
- supporting study
- Study period:
- Not specified
- Reliability:
- 2 (reliable with restrictions)
- Rationale for reliability incl. deficiencies:
- study well documented, meets generally accepted scientific principles, acceptable for assessment
- Study type:
- not specified
- Qualifier:
- no guideline followed
- Principles of method if other than guideline:
- The occurrence of chiral and achiral phenoxyalkanoic acid herbicides in lakes and rivers in Switzerland is reported.
- GLP compliance:
- not specified
- Remarks:
- Publication
- Radiolabelling:
- not specified
- Details on sampling:
- Description of Lakes Sampled.
Surface water was collected from five lakes and two rivers situated in the central and eastern region of Switzerland. The lakes investigated were the Zurichsee (Lake Zurich) and Walensee (sampling near the centers) and the Greifensee, Sempachersee, and Hallwilersee (sampling at outlets). The rivers studied were the Aabach, one of the tributaries to Greifensee (sampling at the inlet to this lake), and the river Reuss (sampling at Ottenbach, ≈34 km from Luzern). Zurichsee (406 m above sea level, msl; two basins, total surface area 88.4 km^2; mean water residence time, 1.2 yr) is situated in a more populated area, although there are still some agricultural activities nearby (catchment area, 1760 km^2; population, 315 000). It receives most of its water from Walensee (419 msl; surface area, 24.1 km^2; mean water residence time, 1.25 yr), which is situated in a more mountainous region. Greifensee is a smaller lake (435 msl; surface area, 8.4 km^2; mean water residence time, 1.1 yr) situated 10 km east of Zurich. There are agricultural activities in its catchment area (160 km^2), but the relatively high population (≈100 000 inhabitants) in this area cause an additional input of anthropogenic compounds. Sempachersee (504 msl; surface area, 14.4 km^2) is situated 40 km southwest of Zurich. This lake has a relatively long mean water residence time of 17 yr. In its relatively small catchment area (61 km^2) are intense agricultural activities, and there is a relatively small population of about 12 000 inhabitants. Finally, Hallwilersee (449 msl; surface area, 10.0 km^2; mean water residence time, 3.8 yr) is situated 25 km southwest of Zurich and has, as does Sempachersee, a predominant input from agricultural activities in its catchment area (138 km^2) (population, 23 000). Typically, these lakes are stratified during the warmer season (April - November) with development of an epilimnion (thermocline at 8 - 15 m) and a hypolimnion. In late fall and winter, the waters are mixed down to significant depths. The residence times for water in the epilimnia during stratification are shorter (5 - 10×) than the mean water residence times indicated above. Lateral mixing in these lakes is fast (within days) as compared to other processes that influence the behavior of the compounds studied. In Sempachersee and Hallwilersee aeration programs are in progress, resulting in total vertical mixing in winter and increased hypolimnion mixing in summer. Additionally, a small mountain lake (Jorisee; 2519 msl; surface area, ≈0.05 km^2) with inputs only from snow, ice, and rain was analysed.
Incubation of Natural and Fortified Lakewater.
Lake water for incubation was taken from Sempachersee on August 2, 1996, and the river Aabach on August 29, 1996. No fortifications were made to these waters. The water from Sempachersee was incubated in 1-L green glass bottles and incubated for up to 129 d at room temperature (rt, 20 - 23 °C). Periodically, one of the bottles was removed from storage, and the water was analysed as described below. The water from the river Aabach was incubated in one batch in a 2.5-L clear glass bottle for up to 50 d at rt while being stirred with a Teflon bar. Periodically, 0.25-L subsamples were removed and analysed as described. From the amounts of oxygen dissolved and the relatively small amounts of dissolved organic matter, it can be assumed that the incubation conditions in both experiments were aerobic. There was some algal growth observed in the clear glass bottle. Additionally, incubation experiments were carried out with fortified water, using water from Sempachersee and the river Aabach, collected on November 4, 1996. Two 2.5-L batches of each were fortified at 50 - 100 ng/L per compound. There was thus one batch each fortified with one R and one S enantiomer of the test material. The waters were incubated at rt in amber bottles for up to 89 d. Periodically, 0.25-L subsamples were removed and analysed as described below. The fortifications were made by adding 1.0 mL of a 250 ng/ mL solution of the compounds in distilled water, prepared from stock solutions of the compounds in methanol.
Sampling and Analytical Procedures.
Surface water (depth, 1 m) from the lakes was collected with standard equipment and filled on-site into methanol-rinsed 1-L glass bottles. A fossil groundwater (zero-contaminant water) was also analysed for control purposes. - Light source:
- not specified
- Reference substance:
- not specified
- Dark controls:
- not specified
- Computational methods:
- Modeling of the Enantiomerisation and Degradation Kinetic
The rate equation for the first-order degradation of an achiral compound is whereby [C] is the concentration and k is the degradation rate of the compound. The rate equations for a chiral compound and the generalised reaction scheme assume first-order kinetics are whereby [R] and [S] are the concentrations, kR and kS are the degradation rates, and kRS (inversion of R to S) and kSR (inversion of S to R) are the enantiomerisation rates of the R and S enantiomers, respectively. For modeling, the derivatives were replaced by finite differences, and a computer program was used to calculate the concentrations of R and S as a function of the rate constants and the time (initial concentrations of the R and S enantiomers were taken as known). The data sets from the incubation experiments were fitted to the above equations using this program and initial estimates of the rates. The rates were then varied in a way to minimise the sum of the squares of the errors. In the experiments where a lag phase was observed the rates were stepped from zero to the full rate at the end of the lag phase in an exponential way, and then varied for a reasonable fit by visual inspection. The model fitted curves are plotted along with some of the data. - Details on results:
- Occurrence of Phenoxyalkanoic Acids and Dicamba in Swiss Lakes and Rivers.
All lakes, with exception of those from the more mountainous areas, contained detectable concentrations of some phenoxyalkanoic acids and dicamba. The concentrations in these lakes were at all times significantly below the ECE drinking water tolerance limit (100 ng/L). Also detected was clofibric acid, a pharmaceutical drug and a close analogue of the test material. The presence of higher concentrations of the test material is not unexpected since it is a mixing partner in numerous herbicide formulations. 2,4,5-T and 2,4,5-TP were not detected (S (ERs up to 4.36) as expected from the soil degradation of the test material. However, Lakes Greifensee and Zurichsee (at particular times) and the rivers Aabach and Reuss unexpectedly showed reversed enantiomeric compositions of S > R (ERs as low as 0.21). ERs < 1 cannot be explained by the soil degradation data and suggest the occurrence of additional, possibly aquatic, enantioselective processes or another route (source) of contamination. Thus, the lakes with the highest contributions from agricultural practices (Sempachersee, Hallwilersee) showed an enantiomeric composition of R > S as expected from the soil degradation data, but some other lakes and rivers did not
Enantiomerisation of the Test Material in Natural Waters.
A sample from Sempachersee, stored for over 2 yr and now analysed also showed a reversed enantiomeric composition (ER = 0.29). Since it is unlikely that the actual test material composition in this lake should be reversed from that of 2 yr earlier, it was hypothesised that the enantiomeric composition of the test material could have changed during storage in the laboratory. To test this hypothesis, incubation experiments with waters from Sempachersee (ER = 2.6; 14 ng/L) and the river Aabach (ER = 0.50; 30 ng/L) were carried out, thus using waters with a normal (R > S) and a reversed (S > R) enantiomeric composition of the test material. The concentrations of the test material, MCPA, 2,4-D, and dicamba were reduced during the 50 - 129 d of incubation in both experiments. The data fitted approximate first-order kinetics without a lag phase. The overall degradation rate constants (k) for the test material (summation of both enantiomers), MCPA, and 2,4-D thus estimated were 2.7 × 10-3, 6.4 × 10-3, and 3.5 × 10-3 d^-1, respectively, using the more precise data from Sempachersee (1-L samples, longer incubation periods), indicating half-lives of 250, 100, and 200 d at 20 - 23 °C, respectively.
The data indicate these compounds to be relatively stable in the aquatic environment whereas they degrade relatively quickly in soil (half-lives, 7 - 22 d). The data from the incubation of water from Sempachersee clearly show enantiomerisation of (R)-test material into (S)-test material. The enantiomeric composition is initially R > S (ER = 2.6) and then gradually changes to a racemic and eventually a reversed composition of S > R (ER = 0.4 after 129 d). In contrast, the data from the incubation of water from the river Aabach showed a relatively constant enantiomeric composition (ER ≈ 0.50). The enantiomeric composition of the test material in both experiments thus was eventually S > R. The data for the test material indicated that the enantiomerisation of R to S is several times faster than that of S to R and faster than actual degradation. The data showed relatively good fits to the first-order model using rate constants kR = 1 × 10^-3 d^-1, kRS = 13.5 × 10^-3 d^-1, kSR = 4.5 × 10^-3 d^-1, and kS = 4.5 × 10^-3 d^-1, as indicated by the model fitted.
Enantiomerisation of the Test Material in Fortified Waters.
The data from the incubation experiments with the fortified waters confirmed that the enantiomerisation of the test material is proceeding predominantly from R to S. The data indicated some initial lag phase (duration 11 - 35 d) with smaller rates that were not observed in the previous experiments and may be due to the different (lower) biotic activity of the waters at this time. The authors plotted the data from an experiment with (R)-test material and (S)-DCPP. The data for the test material show an approximately constant concentration of (R)- test material (55 ng/L; lag phase) up to 21 d, followed by a gradual decrease to ≈11 ng/L at 89 d, the largest decrease occurring between 21 and 63 d. During the latter period, the concentration of (S)-test material gradually increased from ≈3 to 44 - 48 ng/L and then also decreased. The curves for (R)- and (S)-test material thus intersect, leading eventually to a reversed enantiomeric composition (S > R), indicating significant conversion of the R into the S enantiomer.
The authors plotted the data from an experiment with (S)-test material. The data for the test material show a gradual decrease in the concentration of (S)-test material from ≈90 to 48 ng/L at 89 d. There is no increase but rather some decrease of (R)- test material from 16 to 12 ng/L during this time and hence little if any conversion of the S into the R enantiomer.
The results for the test material in all experiments thus indicate significant enantiomerisation of the R into the S enantiomers, but little if any enantiomerisation of the S into the R enantiomers. The authors also plotted model fitted data for the test material using estimates for the various rate constants. The estimates were initially based on the following considerations: (a) the experimental data indicate, after the lag phase, far higher values for kRS than for kSR; (b) at the period of highest change of concentration (21 - 62 d) about 80 % of the decrease in an R enantiomer is converted into the respective S enantiomer, and hence the enantiomerisation rates kRS exceed the respective degradation rates kR; (c) the degradation rates kS (least affected by enantiomerisation), estimated from the data are about 10 × 10^-3 and 8 × 10^-3 d-1 (half-lives, ≈60 and ≈80 d, respectively) for the test material and DCPP, respectively, or less. From these considerations and taking an initial lag phase of 11 - 35 d into account, the rates that gave reasonable fits for the experimental data were kR = 1 × 10^-3 d-1, kRS = 30 × 10^-3 d-1, kSR = 3 × 10^-3 d-1, and kS = 8 × 10^-3 d^-1 for the test material. The rates for the test material estimated in these experiments differed from those of the previous ones with the unfortified “natural” waters, and the modelled data did not fit the experimental data as good. Nevertheless, all data show that kRS and kS dominate over kSR and kR.
Consequences for the Enantiomeric Composition of Environmental Residues of the Test Material.
The various incubation experiments suggest a preference for the S enantiomers in the aquatic environment. Enantiomerisation seems to be a general phenomena as it was observed with waters from Lake Sempachersee and the river Aabach, and at two time periods (August and November 1996). The process is biologically mediated since the inversion of R to S was 3 - 10 × faster than the inversion of S to R. In chemically mediated reactions, the respective enantiomerisation and degradation rates are identical (kR = kS; kRS = kSR), and the degradation curves for the enantiomers do not intersect. The rates reported above should be considered as rough estimates as they likely depend on the actual microbial populations in the waters. The particular kinetic, with the enantiomerisation rates exceeding the degradation rates, leads to a faster dissipation of the R than of the S enantiomer when in fact the degradation rate of the latter enantiomer (kS) is larger (kS > kR). The degradation of the test material in these waters can best be described as an enantiomerisation of the R into the S enantiomer, followed by degradation of the S enantiomer. The curves for (R/S)-test material indicate that the enantiomeric composition remains racemic in case of chemically mediated reactions whereas it is changed to S > R in case of biologically mediated reactions. The curves for (R)- and (S)-test material only intersect in case of biologically mediated reactions.
The enantiomeric composition of the test material in soil from agricultural applications of the registered herbicides is expected to be R > S, but once the compounds are in the aquatic environment, via runoff or leaching from treated areas, and subjected to the processes described here, the composition can be somewhat changed toward the S enantiomer. Two of the lakes, Sempachersee and Hallwilersee, showed test material residues with a composition R > S, as expected from such applications; both lakes have relatively large agricultural activities in their catchment areas. We assume that most of the test material in these lakes is actually from such activities, but apparently the biotic activity and/or the residence time in the lakes are not large enough to fully revert the enantiomeric composition to S > R. The two lakes Greifensee and Zurichsee, and in particular the river Aabach, showed test material residues with enantiomeric compositions of S > R over extended periods of time. ER values as low as 0.21 are difficult to explain from initial compositions of R > S followed by enantiomerisation in the aquatic environment using the rate constants determined in this study. The time required to revert the enantiomeric composition for the test material from R > S to S > R is estimated to be in the order of weeks. While residence times of this magnitude are reached in these lakes, even during stratification, the results from Lake Sempach with the longest residence time still indicate a composition R > S. Furthermore, the river Aabach (inlet to Greifensee) also showed an enantiomeric composition of S > R over a period of several months although the residence time of the test material in the river is expectedly short (hours to days). The situation, however, may be different if one would assume inputs of (R/S)- test material, such as from illegal agricultural applications or from another source. The modelled degradation curves for (R/S)- test material, with the rate estimates of this study, would lead much quicker to enantiomeric compositions of S > R. Since enantiomeric compositions of S > R are particularly observed in lakes with a higher contribution from non-agricultural activities (Greifensee, Zurichsee), such activities may thus be responsible for some of the test material in these lakes. It awaits further investigation whether the recently reported leaching of the test material with runoff water from flat building roofs sealed with bitumenous materials containing (R/S)-test material-polyglycol diesters as a root protectant is such a source. However, because the water flowing out of Lake Greifensee showed a higher ER for the test material than the water in one of the main tributaries to this lake, there must still be other inputs enriched with the R enantiomer such as from agricultural test material applications. For the future, the enantiomeric composition of residues may thus aid in identifying the sources and pathways of contaminations.
After this study, it was found that the test material is present in this particular tributary of the Greifensee only at locations receiving water from wastewater treatment plants, thus pointing to major non-agricultural inputs of test material into this lake. - Validity criteria fulfilled:
- not applicable
- Conclusions:
- The occurrence of chiral and achiral phenoxyalkanoic acid herbicides in lakes and rivers in Switzerland is reported. The compounds most frequently detected were the chiral test material. The compounds were generally present at concentrations well below the ECE recommended drinking water tolerance level (100 ng/L), even in lakes situated in areas with intense agricultural activities. In case of the test material, both enantiomers (R and S) were present, although only the technical material with the R enantiomer is registered and used as a herbicide in Switzerland. Previous studies indicated significant enantiomerisation of the test material in soil leading to residues enriched in R enantiomers independent of whether the racemic (R/S) or the enantiopure R compound was incubated. In some Swiss lakes now, the residues of the test material showed compositions of R > S as expected from the soil degradation data, but in other lakes, surprisingly, “reversed” compositions of S > R were found. This suggested the occurrence of additional biotic processes in the aquatic environment and/or contamination with racemic test material from another source. Laboratory incubation of the test material in lake and river water confirmed significant enantiomerisation. The enantiomerisation is biologically mediated and leads to residues of the test material in these waters, which are eventually enriched in the S enantiomers.
- Executive summary:
The occurrence of chiral and achiral phenoxyalkanoic acid herbicides in lakes and rivers in Switzerland is reported. The compounds most frequently detected were the chiral test material. The compounds were generally present at concentrations well below the ECE recommended drinking water tolerance level (100 ng/L), even in lakes situated in areas with intense agricultural activities. In case of the test material, both enantiomers (R and S) were present, although only the technical material with the R enantiomer is registered and used as a herbicide in Switzerland. Previous studies indicated significant enantiomerisation of the test material in soil leading to residues enriched in R enantiomers independent of whether the racemic (R/S) or the enantiopure R compound was incubated. In some Swiss lakes now, the residues of the test material showed compositions of R > S as expected from the soil degradation data, but in other lakes, surprisingly, “reversed” compositions of S > R were found. This suggested the occurrence of additional biotic processes in the aquatic environment and/or contamination with racemic test material from another source. Laboratory incubation of the test material in lake and river water confirmed significant enantiomerisation. The enantiomerisation is biologically mediated and leads to residues of the test material in these waters, which are eventually enriched in the S enantiomers.
Referenceopen allclose all
Percent Recovery and Percent Test Material Remaining in Aqueous pH 5 Buffer Samples
Matrix | Duration (Hours) | Recovery (%) | TLC Fraction of Test Material (%) | Test Material Remaining (%) |
Non sensitised | 0 | 106 | 98.07 | 104 |
105 | 111 | 91.89 | 102 | |
192 | 108 | 86.79 | 93.7 | |
339 | 102 | 87.98 | 89.7 | |
748 | 97.4 | 64.23 | 62.6 | |
Sensitised (1 % acetone) | 0 | 104 | 97.96 | 102 |
105 | 96.7 | 33.71 | 32.6 | |
154 | 90.7 | 31.99 | 29.0 | |
339 | 77.5 | 4.82 | 3.7 | |
748 | (1) | (1) | (1) | |
Dark Control | 0 | 113 | 96.67 | 109 |
105 | 108 | 97.17 | 105 | |
192 | 104 | 94.09 | 97.9 | |
339 | 109 | 97.39 | 106 | |
748 | 103 | 96.25 | 99.1 |
(1) sample inadvertently unfortified.
Percent Recovery and Percent Test Material Remaining in Aqueous pH 9 Buffer Samples
Matrix | Duration (Hours) | Recovery (%) | TLC Fraction of Test Material (%) | Test Material Remaining (%) |
Non sensitised | 0 | 60.5* | 97.78 | 59.2 |
65.6 | 111 | 93.03 | 103 | |
185 | 109 | 81.97 | 89.3 | |
320 | 07 | 60.01 | 64.2 | |
739 | 96.8 | 56.96 | 55.1 | |
Sensitised (1 % acetone) | 0 | 106 | 97.03 | 103 |
65.6 | 101 | 6975 | 70.4 | |
134 | 103 | 13.41 | 13.9 | |
235 | 97.5 | 3.16 | 3.1 | |
594 | 96.4 | 0.00 | 0.0 | |
Dark Control | 0 | 62.8* | 96.84 | 60.8 |
65.6 | 103 | 96.68 | 99.6 | |
185 | 108 | 96.33 | 104 | |
320 | 117 | 97.65 | 114 | |
739 | 110 | 93.43 | 103 |
*: Low recoveries attributed to inadequate mixing. Values were not used in subsequent calculations.
Duration and Final pH Values for Aqueous pH 5 Buffer Samples
Matrix | Duration (Hours) | Final pH |
Non sensitised | 0 | 5.04 |
105 | 5.02 | |
192 | 5.01 | |
339 | 4.98 | |
748 | 4.96 | |
Sensitised (1 % acetone) | 0 | 5.08 |
105 | 4.96 | |
154 | 5.04 | |
339 | 4.96 | |
748 | 4.84 | |
Dark Control | 0 | 5.04 |
105 | 4.98 | |
154 | 5.00 | |
339 | 5.01 | |
748 | 4.97 |
Initial pH was determined to be 5.00
Duration and Final pH Values for Aqueous pH 7 Buffer Samples
Matrix | Duration (Hours) | Final pH |
Non sensitised | 0 | 6.96 |
105 | 6.92 | |
192 | 6.93 | |
339 | 6.94 | |
748 | 6.85 | |
Sensitised (1 % acetone) | 0 | 6.98 |
105 | 9.89 | |
154 | 6.86 | |
339 | 6.77 | |
748 | 6.43 | |
Dark Control | 0 | 7.00 |
105 | 6.93 | |
192 | 6.96 | |
339 | 6.96 | |
748 | 6.99 |
Initial pH was determined to be 7.00
Duration and Final pH Values for Aqueous pH 9 Buffer Samples
Matrix | Duration (Hours) | Final pH |
Non sensitised | 0 | 8.93 |
65.6 | 8.99 | |
185 | 8.96 | |
320 | 8.93 | |
739 | 8.93 | |
Sensitised (1 % acetone) | 0 | 8.93 |
65.6 | 8.97 | |
134 | 8.96 | |
235 | 8.93 | |
594 | 8.84 | |
Dark Control | 0 | 8.97 |
65.6 | 8.98 | |
185 | 8.97 | |
320 | 8.93 | |
739 | 8.98 |
Initial pH was determined to be 9.01
First Order Treatment of Percentage Test Material Remaining
Sample Description | Y-intercept | Slope | Coefficient of Correlation | Calculated Half Life (Hours) |
pH 5 Non sensitised | 2.03 | -3.01E-04 | -0.98889 | 1 000 |
pH 5 sensitised | 2.02 | -4.20E-03 | 0.99394 | 72 |
pH 5 dark control | 2.02 | -4.03E-05 | -0.58634 | N/C |
pH 7 Non sensitised | 2.04 | -2.84E-04 | -0.99692 | 1 060 |
pH 7 sensitised | 2.10 | -4.28E-03 | -0.98299 | 70 |
pH 7 dark control | 2.00 | -1.99E-06 | -0.05090 | N/C |
pH 9 Non sensitised | 2.01 | -3.91E-04 | -0.92483 | 770 |
pH 9 sensitised | 2.12 | -6.84E-03 | -0.98333 | 44 |
pH 9 dark control | 2.02 | 3.19E-06 | 0.03559 | N/C |
N/C: Half-life not calculated.
Estimation of the Rate of Photolysis of the Test Material in the Preliminary Test
Time (hours) |
Concentration of the Test Material in Solution (mg/L) |
% Applied Test Material (%) |
T0 |
10.47 |
100 |
16.5 |
8.89 |
84.9 |
112.4 |
0.43 |
2.0 |
Concentration of the test Material in Solution Following Irradiation of Sterilised Distilled Water Treated with the Test Material
Exposure |
Sampling Time (Hours) |
Replicate |
Concentration of the Test Material in Solution (mg/L) |
Mean Concentration of the Test Material in Solution (mg/L) |
% Applied Test Material (%) |
Mean % Applied test Material (%) |
|
T0 |
A |
10.03 |
10.06 |
100.82 |
101.14 |
B |
10.10 |
- |
101.46 |
- |
||
Irradiated |
6 |
A |
8.91 |
9.30 |
91.49 |
95.47 |
B |
9.69 |
- |
99.45 |
- |
||
Irradiated |
24 |
A |
7.64 |
7.66 |
76.76 |
76.96 |
B |
7.68 |
- |
77.15 |
- |
||
Irradiated |
51 |
A |
* |
6.88 |
* |
70.60 |
B |
6.88 |
- |
70.60 |
- |
||
Irradiated |
72 |
A |
5.22 |
5.22 |
53.36 |
53.6 |
B |
* |
- |
* |
- |
||
Irradiated |
99 |
A |
3.43 |
3.02 |
34.44 |
30.37 |
B |
2.62 |
- |
26.30 |
- |
||
Irradiated |
120 |
A |
0.92 |
0.89 |
9.22 |
8.98 |
B |
0.87 |
- |
8.75 |
- |
||
Dark control |
48 |
A |
9.99 |
9.99 |
100.45 |
100.45 |
B |
* |
- |
* |
- |
||
Dark control |
120.4 |
A |
9.94 |
9.97 |
99.94 |
100.21 |
B |
10.00 |
- |
100.48 |
- |
*Anomalous results not reported.
Estimation of the Photolytic Degradation Rate and Half-Life of the Test Material in Sterilised Distilled Water.
Time (Hours) |
C0,Ct |
C0/Ct |
1n (C0-Ct) |
T0 |
10.06 |
1.00 |
0.00 |
6 |
9.30 |
1.08 |
0.08 |
24 |
7.66 |
1.31 |
0.27 |
51 |
6.88 |
1.46 |
0.38 |
72 |
5.22 |
1.93 |
0.66 |
99 |
3.02 |
3.33 |
1.20 |
120 |
0.89 |
11.26 |
2.42 |
C0: Initial measured concentration of the test material in the test systems at t0.
Ct: Concentration of the test material in the test system at sampling time t.
For a semi-logarithmic plot of 1n (C0/Ct against time, t:
Slope = 0.01691 h^-1
Intercept = -0.18233 h
Correlation = 0.91567
Rate constant, Kp, is the slope of this line.
Hence Kp = 0.01691 h^-1
DT50 occurs when the chemical reaches one-half of its original concentration. That is:
[Ct] = [C0]/2, t= DT50
At DT50 = 1n 2/Kp
Half-life (DT50) = 0.693 / Kp
DT50 = 41.0 h
Concentration of the Test Material in Solution Following Irradiation of Natural Water Treated with the Test Material
Exposure |
Sampling Time (Hours) |
Replicate |
Concentration of the Test Material in Solution (mg/L) |
Mean Concentration of the Test Material in Solution (mg/) |
% Applied Test Material (%) |
Mean % Applied Test Material (%) |
|
T0 |
A |
10.18 |
10.18 |
99.53 |
99.52 |
B |
10.17 |
- |
99.50 |
- |
||
Irradiated |
6 |
A |
9.64 |
9.54 |
94.64 |
93.65 |
B |
9.44 |
- |
92.66 |
- |
||
Irradiated |
24 |
A |
8.41 |
8.07 |
82.52 |
79.19 |
B |
7.73 |
- |
75.86 |
- |
||
Irradiated |
48 |
A |
6.82 |
6.29 |
66.94 |
61.76 |
B |
5.76 |
- |
56.58 |
- |
||
Irradiated |
72 |
A |
5.76 |
5.06 |
65.53 |
49.68 |
B |
4.36 |
- |
42.83 |
- |
||
Irradiated |
100 |
A |
4.75 |
3.98 |
46.61 |
39.09 |
B |
3.22 |
- |
31.57 |
- |
||
Irradiated |
123 |
A |
3.95 |
3.21 |
38.81 |
31.52 |
B |
2.47 |
- |
24.24 |
- |
||
Irradiated |
170 |
A |
2.77 |
2.11 |
27.20 |
20.71 |
B |
1.45 |
- |
14.21 |
- |
||
Irradiated |
219 |
A |
1.97 |
1.39 |
19.30 |
13.67 |
B |
0.82 |
- |
8.03 |
- |
||
Dark control |
6 |
A |
10.36 |
10.31 |
101.64 |
101.15 |
B |
10.26 |
- |
100.67 |
- |
||
Dark control |
24 |
A |
10.32 |
10.26 |
101.32 |
100.72 |
B |
10.20 |
- |
100.11 |
- |
||
Dark control |
48 |
A |
10.27 |
10.21 |
100.84 |
100.25 |
B |
10.15 |
- |
99.66 |
- |
||
Dark control |
72 |
A |
10.20 |
10.14 |
100.10 |
99.53 |
B |
10.08 |
- |
98.95 |
- |
||
Dark control |
100.5 |
A |
10.20 |
10.15 |
100.14 |
99.59 |
B |
10.09 |
- |
99.04 |
- |
||
Dark control |
124 |
A |
10.22 |
10.14 |
100.36 |
99.52 |
B |
10.05 |
- |
98.69 |
- |
||
Dark control |
170.5 |
A |
10.21 |
10.14 |
100.25 |
99.55 |
B |
10.07 |
- |
98.86 |
- |
||
Dark control |
220 |
A |
10.20 |
10.14 |
100.16 |
99.55 |
B |
10.08 |
- |
98.94 |
- |
Estimation of the Photolytic Degradation Rate and Half-Life of the Test Material in Natural Water
Time (Hours) |
C0,Ct (mg/L) |
C0 / Ct |
1n (C0 /Ct) |
T0 |
10.18 |
1.00 |
0.00 |
6 |
9.54 |
1.07 |
0.06 |
24 |
8.07 |
1.26 |
0.23 |
48 |
6.29 |
1.62 |
0.48 |
72 |
5.06 |
2.01 |
0.70 |
100 |
3.98 |
2.56 |
0.94 |
123 |
3.21 |
3.17 |
1.15 |
170 |
2.11 |
4.82 |
1.57 |
219 |
1.39 |
7.31 |
1.99 |
C0: Initial measured concentration of the test material in the test systems at t0.
Ct: Concentration of the test material in the test system at sampling time t.
For a semi-logarithmic plot of 1n (C0/Ct against time, t:
Slope = 0.00909 h^-1
Intercept = 0.02225 h
Correlation = 0.99967
Rate constant, KP, is the slope of this line. Hence, KP = 0.00909 h^-1
DT50 occurs when the chemical reaches one-half of its original concentration.
That is; [C1] = [C0]/2, t = DT50
At DT50 = 1n 2/Kp
Half-life (DT50) = 0.693 / Kp
DT50 = 76.2 h
Description of key information
Key Study: Crowe (1999)
Under the conditions of the test, the photodegradation of the test material in natural water was shown to follow first-order kinetic behaviour, and the derived photolysis rate constant (Kp) was determined to be 0.0091 hours^-1 and the half-life to be 76.2 hours.
Supporting Study: Meunier & Boule (2000)
The formation of the main primary photoproducts resulting from the phototransformation of the test material is not influenced either by oxygen or by wavelength in the range 254 - 310 nm, but depends on pH (different photochemical behaviour of molecular and anionic forms): Heterolytic photohydrolysis is the main reaction observed with the anionic form, whereas a photochemical rearrangement is the main pathway with the molecular form. A number of other photoproducts were identified: o-cresol, 4-chloro-o-cresol and quinonic derivatives. The photoreactivity of the molecular form is interesting for comparison with other chloroaromatic pesticides, but generally it plays a minor role under environmental conditions.
Different photochemical reactivity was observed when solutions were irradiated in sunlight or nearUV light. Under these conditions 4-chloro-o-cresol is formed as the main photoproduct. This phenomenon confirms the wavelength effect previously reported in the case of 4-chloro-2-methylphenoxyacetic acid. It is attributed to reactions induced by quinonic compounds formed as intermediates.
The transformation of the test material can be induced by Fe(III) salts and nitrite ions, leading to the formation of 4-chloro-2-methylphenol as the main product. An oxidation of the acetic moiety by hydroxyl radicals may be suggested.
The test material does not accumulate in environmental conditions and induced reactions play a major role in the phototransformation.
Supporting Study: Boule et al. (2002)
The photochemical behaviour of the test material depends on both pH and irradiation wavelength.
The pKa of the test material is 3.78. When the anionic form (at pH 5.5) is irradiated at λ ≤ 350 nm photohydrolysis (formation of I) accounts for about 90 % of the transformation. The formation of 2-methylphenol (II) is also reported, but it is a minor pathway. These photoproducts also appear when an aqueous solution is irradiated at pH 2.15 in order to excite the molecular form, but they only account for a low percentage of the transformation. The main product (III) results from a photorearrangement, but some other products are detected, namely 4-chloro-2-methylphenol (IV) resulting from ether bond scission and the substituted quinone (V). In contrast, when an unbuffered solution of the test material is irradiated in sunlight or in near UV light (lamps with a main line at 365 nm and minor emission at 313 and 334 nm), the phototransformation is very slow and the main photoproduct is IV. With the molecular form, disappearance of the test material and formation of IV are reported to be self-accelerated. IV is also the main product when oxidation of the test material is photoinduced by TiO2, nitrite ions, ferric salts or methylbenzoquinone. From these observations it is deduced that formation of IV in sunlight is mainly due to oxidation photoinduced by quinonic derivatives such as product V and methylbenzoquinone.
Supporting Study: Buser & Muller (1998)
The occurrence of chiral and achiral phenoxyalkanoic acid herbicides in lakes and rivers in Switzerland is reported. The compounds most frequently detected were the chiral test material. The compounds were generally present at concentrations well below the ECE recommended drinking water tolerance level (100 ng/L), even in lakes situated in areas with intense agricultural activities. In case of the test material, both enantiomers (R and S) were present, although only the technical material with the R enantiomer is registered and used as a herbicide in Switzerland. Previous studies indicated significant enantiomerisation of the test material in soil leading to residues enriched in R enantiomers independent of whether the racemic (R/S) or the enantiopure R compound was incubated. In some Swiss lakes now, the residues of the test material showed compositions of R > S as expected from the soil degradation data, but in other lakes, surprisingly, “reversed” compositions of S > R were found. This suggested the occurrence of additional biotic processes in the aquatic environment and/or contamination with racemic test material from another source. Laboratory incubation of the test material in lake and river water confirmed significant enantiomerisation. The enantiomerisation is biologically mediated and leads to residues of the test material in these waters, which are eventually enriched in the S enantiomers.
Supporting Study: Obrist (1986)
Under the conditions of the study, degradation of the test material in aqueous buffer solutions was photolytically driven. The half-life was calculated to be 72, 70, and 44 hours in sensitised aqueous buffer at pH 5, 7, and 9, respectively. In non-sensitised aqueous buffer, the calculated half-life was 1 000, 1 060, and 770 hours at pH 5, 7, and 9, respectively. Two major photoproducts (greater than 10 % of applied radioactivity) and several minor products were formed in some of the sensitised and non-sensitised samples. Because of the further separation of radioactive components in this investigation, the degradation half-lives of 14C-test material were recalculated to be: 680 (pH 5), 1 019 (pH 7), and 415 (pH 9) hours.
Key value for chemical safety assessment
- Half-life in water:
- 76.2 h
Additional information
Key Study: Crowe (1999)
The photolysis rate constant and half-life of the test material in sterile distilled and natural water at 25 °C were assessed in order to meet the requirements of JMAFF Guidelines and Section 7.2.1.2 of Annex II of Directive 91/414/EEC.
The study was awarded a reliability score of 1 in accordance with the criteria set forth by Klimisch et al. (1997).
The ultraviolet/visible absorption spectrum of the test material showed a wavelength maximum (λmax) at 290 nm. The absorbance at 290 nm was 0.032 AU for a solution of 9.7 mg/L. On this basis the test solution concentration for the test material (less than half-saturation and an absorbance of less than 0.05 AU at wavelengths greater than 290 nm) was selected to be 10 mg/L.
A preliminary photodegradation test was performed at 25 °C, by exposing cells containing the test material in distilled water to artificial sunlight (provided by a xenon arc lamp set at 425 W m^-2 inside the Heraeus Suntest CPS exposure unit). The test material was shown to degrade such that 2.0 % of the applied test material remained in solution after 112.4 hours.
On the basis of the results from the preliminary test, the photodegradation of the test material in sterile distilled water test was conducted with samples taken at T0, 6, 24, 51, 72, 99 and 120 hours. Hydrolytic stability of the test material was shown by the stability of the test material in dark controls. This allowed direct determination of the rate of photodegradation of the test material in solution. Photodegradation of the test material in sterile distilled water was shown to follow first-order kinetic behaviour, and the derived photolysis rate constant (Kp) was determined to be 0.0169 hours^-1 and the half-life to be 41.0 hours at 25 °C.
The photodegradation of the test material in natural water test was conducted with samples taken at T0, 6, 24, 48, 72, 100, 123, 170 and 219 hours. Biotic stability of the test material was shown by the absence of degradation of the test material in the dark controls. This allowed direct determination of the rate of photodegradation of the test material in solution.
Under the conditions of the test, the photodegradation of the test material in natural water was shown to follow first-order kinetic behaviour, and the derived photolysis rate constant (Kp) was determined to be 0.0091 hours^-1 and the half-life to be 76.2 hours.
Supporting Study: Meunier & Boule (2000)
The test material was irradiated under various conditions of pH, oxygenation and wavelengths in order to study the reactions involved in the phototransformation. The study was awarded a reliability score of 2 in accordance with the criteria set forth by Klimisch et al. (1997).
Four main photoproducts were identified: 2-(4-hydroxy-2-methylphenoxy)propionic acid, o-cresol, 2-(5-chloro-2-hydroxy-3- methylphenyl)propionic acid, and 4-chloro-o-cresol. When the anionic form of the test material was irradiated between 254 nm and 310 nm (UV-C or UV-B), 2-(4-hydroxy-2-methylphenoxy)propionic acid was the main photoproduct. At 254 nm its formation initially accounted for more than 80 % of the transformation. The reaction results from a heterolytic photohydrolysis. O-cresol accounted for only a low percentage of the transformation. The stoichiometry was different with the molecular form: The main photoproduct, 2-(5-chloro-2-hydroxy-3- methylphenyl)propionic acid, resulted from a rearrangement after a homolytic scission. Products 2-(4-hydroxy-2-methylphenoxy)propionic acid, o-cresol and 4-chloro-o-cresol were also formed as minor photoproducts. Some other minor photoproducts were also identified. In contrast, 4-chloro-o-cresol was the main photoproduct under sunlight irradiation or when solutions were irradiated in near-UV light (UV-A). This wavelength effect is attributed to the involvement of an induced phototransformation; 4-chloro-o-cresol is also the main photoproduct when the phototransformation is induced by Fe(III) perchlorate or nitrite ions. In usual environmental conditions the excitation of the molecular form is negligible and the phototransformation is mainly due to induced photoreactions.
Supporting Study: Boule et al. (2002)
The study investigated the direct phototransformation of aromatic derivatives. The study was awarded a reliability score of 2 in accordance with the criteria set forth by Klimisch et al. (1997).
The photochemical behaviour of the test material depends on both pH and irradiation wavelength.
The pKa of the test material is 3.78. When the anionic form (at pH 5.5) is irradiated at λ ≤ 350 nm photohydrolysis (formation of I) accounts for about 90 % of the transformation. The formation of 2-methylphenol (II) is also reported, but it is a minor pathway. These photoproducts also appear when an aqueous solution is irradiated at pH 2.15 in order to excite the molecular form, but they only account for a low percentage of the transformation. The main product (III) results from a photorearrangement, but some other products are detected, namely 4-chloro-2-methylphenol (IV) resulting from ether bond scission and the substituted quinone (V). In contrast, when an unbuffered solution of the test material is irradiated in sunlight or in near UV light (lamps with a main line at 365 nm and minor emission at 313 and 334 nm), the phototransformation is very slow and the main photoproduct is IV. With the molecular form, disappearance of the test material and formation of IV are reported to be self-accelerated. IV is also the main product when oxidation of the test material is photoinduced by TiO2, nitrite ions, ferric salts or methylbenzoquinone. From these observations it is deduced that formation of IV in sunlight is mainly due to oxidation photoinduced by quinonic derivatives such as product V and methylbenzoquinone.
Supporting Study: Buser & Muller (1998)
The occurrence of chiral and achiral phenoxyalkanoic acid herbicides in lakes and rivers in Switzerland is reported. The study was awarded a reliability score of 2 in accordance with the criteria set forth by Klimisch et al. (1997).
The compounds most frequently detected were the chiral test material. The compounds were generally present at concentrations well below the ECE recommended drinking water tolerance level (100 ng/L), even in lakes situated in areas with intense agricultural activities. In case of the test material, both enantiomers (R and S) were present, although only the technical material with the R enantiomer is registered and used as a herbicide in Switzerland. Previous studies indicated significant enantiomerisation of the test material in soil leading to residues enriched in R enantiomers independent of whether the racemic (R/S) or the enantiopure R compound was incubated. In some Swiss lakes now, the residues of the test material showed compositions of R > S as expected from the soil degradation data, but in other lakes, surprisingly, “reversed” compositions of S > R were found. This suggested the occurrence of additional biotic processes in the aquatic environment and/or contamination with racemic test material from another source. Laboratory incubation of the test material in lake and river water confirmed significant enantiomerisation. The enantiomerisation is biologically mediated and leads to residues of the test material in these waters, which are eventually enriched in the S enantiomers.
Supporting Study: Obrist (1986)
The phototransformation in water of the test substance was assessed according to EPA Guideline Subdivision N 161-2 and according to the principles of GLP. The study was awarded a reliability score of 2 in accordance with the criteria set forth by Klimisch et al. (1997).
Spectro-cells and glass vials that contained sterile solutions of test material buffered at pH 5, 7, and 9 were prepared. One set of cells was fortified with acetone to sensitise the solution, and another set of cells remained non-sensitised. One set of glass vials was wrapped in aluminium foil and placed with the cells for dark controls. The cells and vials were exposed to Chroma 50® artificial sunlight. They constituted the photolysis portion of the study. At various times cells and vials were removed and analysed for evidence of degradation of the test material. Photolytic degradation of the test material occurred in both the sensitised and non-sensitised aqueous buffer solutions.
Under the conditions of the study, degradation of the test material in aqueous buffer solutions was photolytically driven. The half-life was calculated to be 72, 70, and 44 hours in sensitised aqueous buffer at pH 5, 7, and 9, respectively. In non-sensitised aqueous buffer, the calculated half-life was 1 000, 1 060, and 770 hours at pH 5, 7, and 9, respectively. Two major photoproducts (greater than 10 % of applied radioactivity) and several minor products were formed in some of the sensitised and non-sensitised samples. Because of the further separation of radioactive components in this investigation, the degradation half-lives of 14C-test material were recalculated to be: 680 (pH 5), 1 019 (pH 7), and 415 (pH 9) hours.
Information on Registered Substances comes from registration dossiers which have been assigned a registration number. The assignment of a registration number does however not guarantee that the information in the dossier is correct or that the dossier is compliant with Regulation (EC) No 1907/2006 (the REACH Regulation). This information has not been reviewed or verified by the Agency or any other authority. The content is subject to change without prior notice.
Reproduction or further distribution of this information may be subject to copyright protection. Use of the information without obtaining the permission from the owner(s) of the respective information might violate the rights of the owner.
