Registration Dossier
Registration Dossier
Data platform availability banner - registered substances factsheets
Please be aware that this old REACH registration data factsheet is no longer maintained; it remains frozen as of 19th May 2023.
The new ECHA CHEM database has been released by ECHA, and it now contains all REACH registration data. There are more details on the transition of ECHA's published data to ECHA CHEM here.
Diss Factsheets
Use of this information is subject to copyright laws and may require the permission of the owner of the information, as described in the ECHA Legal Notice.
EC number: 231-388-1 | CAS number: 7526-26-3
- Life Cycle description
- Uses advised against
- Endpoint summary
- Appearance / physical state / colour
- Melting point / freezing point
- Boiling point
- Density
- Particle size distribution (Granulometry)
- Vapour pressure
- Partition coefficient
- Water solubility
- Solubility in organic solvents / fat solubility
- Surface tension
- Flash point
- Auto flammability
- Flammability
- Explosiveness
- Oxidising properties
- Oxidation reduction potential
- Stability in organic solvents and identity of relevant degradation products
- Storage stability and reactivity towards container material
- Stability: thermal, sunlight, metals
- pH
- Dissociation constant
- Viscosity
- Additional physico-chemical information
- Additional physico-chemical properties of nanomaterials
- Nanomaterial agglomeration / aggregation
- Nanomaterial crystalline phase
- Nanomaterial crystallite and grain size
- Nanomaterial aspect ratio / shape
- Nanomaterial specific surface area
- Nanomaterial Zeta potential
- Nanomaterial surface chemistry
- Nanomaterial dustiness
- Nanomaterial porosity
- Nanomaterial pour density
- Nanomaterial photocatalytic activity
- Nanomaterial radical formation potential
- Nanomaterial catalytic activity
- Endpoint summary
- Stability
- Biodegradation
- Bioaccumulation
- Transport and distribution
- Environmental data
- Additional information on environmental fate and behaviour
- Ecotoxicological Summary
- Aquatic toxicity
- Endpoint summary
- Short-term toxicity to fish
- Long-term toxicity to fish
- Short-term toxicity to aquatic invertebrates
- Long-term toxicity to aquatic invertebrates
- Toxicity to aquatic algae and cyanobacteria
- Toxicity to aquatic plants other than algae
- Toxicity to microorganisms
- Endocrine disrupter testing in aquatic vertebrates – in vivo
- Toxicity to other aquatic organisms
- Sediment toxicity
- Terrestrial toxicity
- Biological effects monitoring
- Biotransformation and kinetics
- Additional ecotoxological information
- Toxicological Summary
- Toxicokinetics, metabolism and distribution
- Acute Toxicity
- Irritation / corrosion
- Sensitisation
- Repeated dose toxicity
- Genetic toxicity
- Carcinogenicity
- Toxicity to reproduction
- Specific investigations
- Exposure related observations in humans
- Toxic effects on livestock and pets
- Additional toxicological data
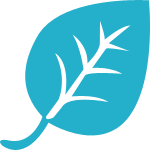
Additional information on environmental fate and behaviour
Administrative data
- Endpoint:
- additional information on environmental fate and behaviour
- Type of information:
- other: Experimental result of Metabolite: MPA (Methylphosphonic acid, CAS 993-13-5)
- Adequacy of study:
- key study
- Reliability:
- 1 (reliable without restriction)
- Rationale for reliability incl. deficiencies:
- other: Publication about an investigation of a metabolite of the registered substance. No standard procedure is used, but scientifically validity criteria are met and described in detail.
Data source
Reference
- Reference Type:
- publication
- Title:
- Unnamed
- Year:
- 2 002
Materials and methods
- Principles of method if other than guideline:
- Summary of phosphonate degradation by different microorganisms via several pathways.
- GLP compliance:
- not specified
- Remarks:
- Publication about several experiments.
- Type of study / information:
- Degradation of phosphonates by procaryotic microorganisms and lower eucaryotes.
Test material
- Reference substance name:
- Methylphosphonic acid (MPA), Metabolite of Diphenyl methylphosphonate (DPP)
- IUPAC Name:
- Methylphosphonic acid (MPA), Metabolite of Diphenyl methylphosphonate (DPP)
- Reference substance name:
- Methylphosphonic acid
- EC Number:
- 213-607-2
- EC Name:
- Methylphosphonic acid
- Cas Number:
- 993-13-5
- Molecular formula:
- CH5O3P
- IUPAC Name:
- methylphosphonic acid
- Test material form:
- not specified
- Details on test material:
- No details available.
Constituent 1
Constituent 2
Results and discussion
Any other information on results incl. tables
Phosphonate-degrading microorganisms are widely spread in nature, including Gram-positive as well as Gram-negative bacteria, some yeasts and fungi. However, only special strains are capable of phosphonate degradation. Gram-positive bacteria are able to directly degrade the C-P bond.
Gram-negative bacteria use the C-P lyase for this degradation mode, only two Gram-positive bacteria are known, using the same enzyme: Arthrobacter sp. and B. megaterium. In fungi, no C-P lyase activity was found.
It is not important, if the phosphonates are of natural or xenobiotic origin, the mineralization is not influenced by the source. The first evidence of biological cleavage of the C-P bond in phosphonates was obtained in Escherichia coli, which utilizes i.e. methylphosphonic acid as sole phosporous source.
The main phenomenon of degradation of methylphosphonic acid by E.coli cells is predominant formation of methane as the final reaction product in a ratio of 1:1 to intracellular phosphorus formed from alkylphosphonates. Also alkenes, not only alkanes are found among the degradation pathway, however, the final phosphorous product is yet unclear.
Concerning the genetic characterisation of the phosphonate degradation, mapping and molecular cloning of the phn genes, which are responsible for the C-P lyase activity, have been performed in E.coli. Three gene products (PhnC, PhnD, and PhnE) are constituents of the alkylphosphonate transporter. Seven gene products (PhnG - PhnM) participate in the catalysis of degradation of alkylphosphonates and are components of membrane associated C-P lyase complex. PhnN and PhnP, two further gene products, are not required for phosphonate use and may be accessory proteins. PhnF and PhnO are unnecessary for the catalysis and are detected to play a role in regulatory proteins. The genes are induced under phosphorous starvation. The strains of E.coli K-12 grow on a medium with methylphosphonic acid (MPA) as the sole source of phosphorus when a spontaneous activation of the phn operon takes place. Homologous genes have also been found in Rhizobium melitoti.
Under anaerobic conditions, biodegradation of phosphonates was shown in Rhodobacter capsulatus, a photosynthetic bacterium.
However, the role of aeration is not clear, since in some E.coli strains, MPA degradation was inhibited by free oxygen, while in others microaerobic conditions are required for this process and free nitrogen is an inhibitor.
Applicant's summary and conclusion
- Conclusions:
- Publication about the degradation potential of Phosphonates, i.e. Methylphosphonic Acid (MPA), which is a metabolite of Diphenyl methylphosphonate (DPP), by different procaryotic microorganisms.
- Executive summary:
Phosphonates occur widely in nature, also by mankind sources (xenobiotics) and are characterized by the chemically inert carbon-to-phosphorus (C-P) bond. They are resistant to chemical hydrolysis and thermal decomposition. Only lower eucyryotic and procaryotic microorganisms are capable to biodegrade phosphonates via several pathways. Kononova and Nesmeyanova (2002) summarized several experimental results about this topic in their paper "Phosphonates and Their Degradation by Microorganisms". The first evidence of biological cleavage of the C-P bond was obtained with Escherichia coli (E.coli) which uses inter alia methylphosphonic acid (MPA) as sole phosphorous source. MPA was detected as a metabolite of the registered substance Diphenyl methylphosphonate (DPP). A wide occurrence of phosphonate-degrading microorganisms in nature is known, including Gram-positive and Gram-negative bacteria as well as some yeasts and fungi. However, only special strains are capable of phosphonate degradation. Gram-positive bacteria are able to degrade the C-P bond directly. Both, natural and xenobiotic phosphonates can be mineralized. Over the time, the following enzymes were detected in different microorganisms, able to cleave the C-P bond: phosphonatase, phosphonoacetate hydrolase, phosphonopyruvate hydrolase and C-P lyase.
In E.coli, the main phenomenon of degradation of MPA is the formation of methane as final reaction product in a ratio of 1:1 to intracellular phosphorus formed from alkylphosphonates. The radical-based mechanisms is confirmed by the formation of alkenes as well. However, the final phosphorous product is not yet identified.
The C-P lyase pathway was also detected in the phototrophic bacterium Rhodobacter capsulatus. Concerning Gram-positive bacteria, only two representatives showed C-P lyase activity: Arthrobacter sp. and Bacillus magaterium. No enzyme activity was found in fungi. Thus, the ability to degrade phosphonates via the C-P lyase mechanism occurs mostly among Gram-negative bacteria.
The degradation pathway was genetically characterized within E.coli, detecting that the phn genes are responsible for the C-P lyase activity. The following conclusion were established: Three gene products (PhnC, PhnD, and PhnE) are constituents of the alkylphosphonate transporter. Seven gene products (PhnG - PhnM) participate in the catalysis of degradation and are components of the membrane associated C-P lyase complex. PhnN and PhnP are not required for the phosphonate use and may be accessory proteins for the enzyme. PhnN may possess also ATPase activity. PhnF and PhnO play a regulatory role only. The genes of the phn operon are induced under phosphorus starvation. The strains of E.coli K-12 are able to grow on medium with MPA as sole source of phosphorus when a spontaneous activation of the phn operon takes place.
Homologous genes were found in Rhizobium meliloti (soil bacteria which are able to fix nitrogen). The bacterium can be distinguished from E.coli by its broader substrate specificity, which is similar to those of Agrobacter radiobacter and Arthrobacter sp. However, these species use two different C-P lyases, whereas Rhiz. meliloti uses only one.
The phosphonate biodegradation process under anaerobic conditions was shown first in the photosynthetic bacterium Rhodobacter capsulatus. In some E.coli strains, the degradation of MPA is inhibited by freely available oxygen, while in others microaerobic conditions are required and free nitrogen inhibits the process. Thus, the role of aeration is not yet clarified at all.
Information on Registered Substances comes from registration dossiers which have been assigned a registration number. The assignment of a registration number does however not guarantee that the information in the dossier is correct or that the dossier is compliant with Regulation (EC) No 1907/2006 (the REACH Regulation). This information has not been reviewed or verified by the Agency or any other authority. The content is subject to change without prior notice.
Reproduction or further distribution of this information may be subject to copyright protection. Use of the information without obtaining the permission from the owner(s) of the respective information might violate the rights of the owner.
