Registration Dossier
Registration Dossier
Data platform availability banner - registered substances factsheets
Please be aware that this old REACH registration data factsheet is no longer maintained; it remains frozen as of 19th May 2023.
The new ECHA CHEM database has been released by ECHA, and it now contains all REACH registration data. There are more details on the transition of ECHA's published data to ECHA CHEM here.
Diss Factsheets
Use of this information is subject to copyright laws and may require the permission of the owner of the information, as described in the ECHA Legal Notice.
EC number: 473-390-7 | CAS number: -
- Life Cycle description
- Uses advised against
- Endpoint summary
- Appearance / physical state / colour
- Melting point / freezing point
- Boiling point
- Density
- Particle size distribution (Granulometry)
- Vapour pressure
- Partition coefficient
- Water solubility
- Solubility in organic solvents / fat solubility
- Surface tension
- Flash point
- Auto flammability
- Flammability
- Explosiveness
- Oxidising properties
- Oxidation reduction potential
- Stability in organic solvents and identity of relevant degradation products
- Storage stability and reactivity towards container material
- Stability: thermal, sunlight, metals
- pH
- Dissociation constant
- Viscosity
- Additional physico-chemical information
- Additional physico-chemical properties of nanomaterials
- Nanomaterial agglomeration / aggregation
- Nanomaterial crystalline phase
- Nanomaterial crystallite and grain size
- Nanomaterial aspect ratio / shape
- Nanomaterial specific surface area
- Nanomaterial Zeta potential
- Nanomaterial surface chemistry
- Nanomaterial dustiness
- Nanomaterial porosity
- Nanomaterial pour density
- Nanomaterial photocatalytic activity
- Nanomaterial radical formation potential
- Nanomaterial catalytic activity
- Endpoint summary
- Stability
- Biodegradation
- Bioaccumulation
- Transport and distribution
- Environmental data
- Additional information on environmental fate and behaviour
- Ecotoxicological Summary
- Aquatic toxicity
- Endpoint summary
- Short-term toxicity to fish
- Long-term toxicity to fish
- Short-term toxicity to aquatic invertebrates
- Long-term toxicity to aquatic invertebrates
- Toxicity to aquatic algae and cyanobacteria
- Toxicity to aquatic plants other than algae
- Toxicity to microorganisms
- Endocrine disrupter testing in aquatic vertebrates – in vivo
- Toxicity to other aquatic organisms
- Sediment toxicity
- Terrestrial toxicity
- Biological effects monitoring
- Biotransformation and kinetics
- Additional ecotoxological information
- Toxicological Summary
- Toxicokinetics, metabolism and distribution
- Acute Toxicity
- Irritation / corrosion
- Sensitisation
- Repeated dose toxicity
- Genetic toxicity
- Carcinogenicity
- Toxicity to reproduction
- Specific investigations
- Exposure related observations in humans
- Toxic effects on livestock and pets
- Additional toxicological data
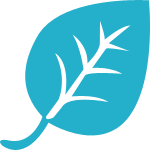
Henry's Law constant
Administrative data
Link to relevant study record(s)
Description of key information
Key value for chemical safety assessment
- Henry's law constant (H) (in Pa m³/mol):
- 104 000 000
- at the temperature of:
- 22 °C
Additional information
The dimensionless Henry’s Law Constant (HLC) was measured experimentally by analytically quantifying the concentration of FC770 in both the headspace (concentration low ppm) and the water phase (low ppb) of a sealed vial after equilibration. The resulting measured HLC of 42,400 (dimensionless, expressed as Cg/Cw, 2.36e-5 expressed as Cw/Cg, or 1030 expressed as atm∙m³/mole) is rather high given the rather low vapor pressure 50.6 mm Hg. However, this HLC is well within the range of many super hydrophobic chemistries and halogenated aromatics.(1)
The HLC for a molecule is simply related to the free energy of hydration (deltaGhyd)(1), which in turn can be considered as the sum of the energy necessary to rearrange a molecule into the optimum conformation for solvation (deltaGstructure) and the energy required to rearrange the solvent in such a way that it will solvate the molecule (deltaGsolv). The equation below provides the exact thermodynamic definitions:
deltaGhyd = deltaGstructure + deltaGsolvent = -RT∙ln(Cw/Cg)
where (Cw/Cg) is the dimensionless HLC and temperature is in Kelvin. Solving the equation above for FC-770 gives a deltaGhyd = 26.1 KJ/mole. The experimentally determined deltaGstructure energy for the optimized structure of 2,2,3,3,5,5,6,6-octafluro-4-(trifluoromethyl)morpholine in water is -4.04 kJ/mole.(2) This structure differs from FC-770 by a perfluoromethyl functionality as opposed to a perfluoropropyl functionality in FC-770. As the two are structurally very similar, the structural rearrangement energy is likely very close. Therefore, in this argument, a deltaGsolv for FC-770 of 30 KJ/mole is used for illustrative purposes. The sign of the energy of solvation is positive, indicating that energy must be added to the system to get FC-770 in solution. This is approximately the same amount of energy as is released in the body through hydrolysis of the high-energy phosphate bond in ATP:
ATP4- + H2O → ADP3- + HPO42- + H+ (30.5 kJ/mol).(3)
Quite simply, this energy is in the range of bond cleavage and it is not spontaneous. Solvation of FC-770 is not favored, just as spontaneous formation of ATP from ADP and phosphate is not favored. Alternatively, the solvation energy is roughly equivalent to the energy required to break five hydrogen bonds while leaving the involved water molecules in place in the bulk solution (ca. 6 kJ/mol).(4) This can be viewed as the energy of forming a pocket in the bulk solution and optimizing it to fit the FC-770 molecule. Upon desolvation of the FC-770 molecule the stored solvation energy is released, forming a thermodynamic driver to remove FC-770 from solution. This provides key thermodynamic evidence for the net removal of FC-770 from open systems.
For environmental fate, FC-770 released to the air will predominantly stay in the air as it is highly energetically unfavorable for FC-770 to be in the water. This is true even for cold water as the temperature dependence of Henry's Law can be regarded as constant over the relatively narrow range from 22 °C to 0 °C. The value of deltaGhyd is 24.2 kJ/mol at the lower temperature. Release of FC-770 from the water to the air is always favored.
There could be some potential for FC-770 to be trapped in ice, particularly in high altitude cirrus clouds or in atmospheric gas bubbles entrapped in ice. In general, one can classify ice - trace gas interactions of three different kinds. One is the partitioning of gases to the ice, leading to a (temporary) loss from the gas phase. This is not an incision or entrapment mechanism. The second is a chemical reaction with the ice (e.g. acids that deprotonate form a volatile neutral molecule to a non-volatile anion and cation). The third is chemical reaction on the ice while the species of interest is partitioned to the ice surface. Temporary distribution to snow and is not favored. Sorption to snow surfaces can be described by a Linear Free Energy Relationship involving two hydrogen bonding terms and the hexadecane-air distribution coefficient (L16) to account for non-specific intermolecular forces.(5) Since FC-770 does not participate in hydrogen bonding, the relationship is limited to log Ksnow/air = 0.639*log L16 - 6.85 at -6.8 °C. The log L16 value is expected to be similar to the log Koa of 1.5, therefore the log Ksnow/air should be approximately -5. It is evident from this that sorption to snow surfaces is not favored for FC-770. A similar relationship describes sorption to liquid water surfaces at 15 °C, Kws/air = 0.635 log L16 - 8.47 (5), indicating that liquid water surfaces at higher temperatures are even less likely to retain FC-770. FC-770 does not react with ice so that will not be a mechanism of transport. In the unlikely event of photolytic transformation of FC-770 on ice surfaces, it would be the photodegradation products that would be transported to the surface and not FC-770. We note that gas bubble encapsulation may occur and result in transport of FC-770 to the terrestrial compartment, this transport is as a gas in air and once the entrapped gas is released, FC-770 will remain in the gaseous state.
Based on a high Log Koc, it could be postulated that FC-770 might adsorb to suspended particulate matter and thereby be transport via atmospheric settling or rain out to the terrestrial surface. It should be noted that the suspended particulate matter is coated with water and is often the source of nucleation for formation of aerosols and water droplets. It is not a significant source of ice formation. Once the suspended particulate begins to add additional water, FC-770 will be driven off by the depositing water. Atmospheric deposition of FC-770 beyond simple diffusion is not an important fate process.
1. Schuurmann, G. 2000. Prediction of Henry’s law constant of benzene derivatives using chemical continuum-solvation models. J. Comp. Chem., 21 (1): 17-34
2. Spartan Parallel Suit Database. (Available to users with a license to use Spartan.) Spartan is a molecular modeling software which uses theoretical quantum mechanical models to calculate physical chemical properties.
3. Lubert Stryer Biochemistry, 3rd edition, 1988. Chapter 13, p. 318
4. Martin Chaplin. 2019. Water Structure and Science: Hydrogen Bonding in water (1). http://www1.lsbu.ac.uk/water/water_hydrogen_bonding.html
5. Lei Y.D., Wania, F. 2004. Is rain or snow a more efficient scavenger of organic chemicals? Atm. Environ., 38(22): 3557-3571.
Information on Registered Substances comes from registration dossiers which have been assigned a registration number. The assignment of a registration number does however not guarantee that the information in the dossier is correct or that the dossier is compliant with Regulation (EC) No 1907/2006 (the REACH Regulation). This information has not been reviewed or verified by the Agency or any other authority. The content is subject to change without prior notice.
Reproduction or further distribution of this information may be subject to copyright protection. Use of the information without obtaining the permission from the owner(s) of the respective information might violate the rights of the owner.
