Registration Dossier
Registration Dossier
Data platform availability banner - registered substances factsheets
Please be aware that this old REACH registration data factsheet is no longer maintained; it remains frozen as of 19th May 2023.
The new ECHA CHEM database has been released by ECHA, and it now contains all REACH registration data. There are more details on the transition of ECHA's published data to ECHA CHEM here.
Diss Factsheets
Use of this information is subject to copyright laws and may require the permission of the owner of the information, as described in the ECHA Legal Notice.
EC number: 700-903-6 | CAS number: 255830-15-0
- Life Cycle description
- Uses advised against
- Endpoint summary
- Appearance / physical state / colour
- Melting point / freezing point
- Boiling point
- Density
- Particle size distribution (Granulometry)
- Vapour pressure
- Partition coefficient
- Water solubility
- Solubility in organic solvents / fat solubility
- Surface tension
- Flash point
- Auto flammability
- Flammability
- Explosiveness
- Oxidising properties
- Oxidation reduction potential
- Stability in organic solvents and identity of relevant degradation products
- Storage stability and reactivity towards container material
- Stability: thermal, sunlight, metals
- pH
- Dissociation constant
- Viscosity
- Additional physico-chemical information
- Additional physico-chemical properties of nanomaterials
- Nanomaterial agglomeration / aggregation
- Nanomaterial crystalline phase
- Nanomaterial crystallite and grain size
- Nanomaterial aspect ratio / shape
- Nanomaterial specific surface area
- Nanomaterial Zeta potential
- Nanomaterial surface chemistry
- Nanomaterial dustiness
- Nanomaterial porosity
- Nanomaterial pour density
- Nanomaterial photocatalytic activity
- Nanomaterial radical formation potential
- Nanomaterial catalytic activity
- Endpoint summary
- Stability
- Biodegradation
- Bioaccumulation
- Transport and distribution
- Environmental data
- Additional information on environmental fate and behaviour
- Ecotoxicological Summary
- Aquatic toxicity
- Endpoint summary
- Short-term toxicity to fish
- Long-term toxicity to fish
- Short-term toxicity to aquatic invertebrates
- Long-term toxicity to aquatic invertebrates
- Toxicity to aquatic algae and cyanobacteria
- Toxicity to aquatic plants other than algae
- Toxicity to microorganisms
- Endocrine disrupter testing in aquatic vertebrates – in vivo
- Toxicity to other aquatic organisms
- Sediment toxicity
- Terrestrial toxicity
- Biological effects monitoring
- Biotransformation and kinetics
- Additional ecotoxological information
- Toxicological Summary
- Toxicokinetics, metabolism and distribution
- Acute Toxicity
- Irritation / corrosion
- Sensitisation
- Repeated dose toxicity
- Genetic toxicity
- Carcinogenicity
- Toxicity to reproduction
- Specific investigations
- Exposure related observations in humans
- Toxic effects on livestock and pets
- Additional toxicological data
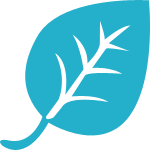
Endpoint summary
Administrative data
Description of key information
Additional information
- Sewage treatment plants
- Water
- Soil
Biodegradation of phosphonates by pure cultures
The bacterial degradation of phosphonic acids and phosphonates has been studied. The C-P bond confers molecular stability to phosphonates, which are, as a result, resistant to chemical, photolytic and thermal decomposition. However, there are several laboratory studies that report degradation of phosphonates by pure microbial cultures in the absence of other phosphorus sources. Species able to utilise phosphonate include Bacillus, Pseudomonas, Arthobacter, Escherischia, Actinomyces, Rhizobium, Acinetobacter, Alcaligenes and Klebsiella. Phosphonate utilisation in many microorganisms is suppressed by orthophosphate. Arthobacter cleaves only a single phosphonate group from ATMP (Gledhill and Feijtel (1992).
Biodegradation of phosphonates by natural populations
Many studies with natural populations show little or no biodegradation of phosphonates in biodegradation tests, although bacteria able to degrade phosphonates are found in both polluted and unpolluted samples. The limiting factors in degradation of phosphonates by environmental samples include numbers of bacteria, bioavailability, and environmental conditions.
The lack of biodegradation in screening tests may be a result of the presence of inorganic phosphorus in test systems. The enzyme needed to enable bacteria to degrade phosphonates is one that is not present unless the bacteria have been in an environment where low orthophosphorus conditions limit growth. In these conditions, the necessary enzyme is induced, and then phosphonates can be metabolised as a source of phosphorus. Once induced, the enzyme is not suppressed by the presence of phosphate, at low concentrations (McGrath 1997).
Where phosphonate concentrations are high, phosphonates are expected to serve as a carbon source (Zahn-Wellens test for ATMP result of 33% DOC removal (Steber and Wierich, (1987)), but studies of ATMP and structurally analogous phosphonates indicated that they are not used as a phosphorus or carbon and energy source (McGrath 1997 and SafePharm 2002).
Biodegradation in specific environments
Very low levels of biodegradation of ATMP-N-oxide were seen in screening studies using mixed cultures. Studies of removal of phosphonates from sewage treatment plants that distinguish between adsorption and biodegradation indicate that phosphonates are resistant to biological degradation during conventional wastewater treatments (Nowack and Stone (2000)).
The variety of laboratory experiments using activated sludge sampled from waste water treatment plants in studies of aerobic biodegradability demonstrate a range of results. Once effects of adsorption in the test system is eliminated, an insignificant level of degradation is observed overall, even in SCAS tests, and in tests using pre-acclimatised organisms.
There are few reports of anaerobic degradation in sewage treatment plants. A study of HEDP and ATMP indicated that their anaerobic degradation in a model digester is low (Gledhill and Feijtel (1992) citing Steber and Wierich, (1986 and 1987). They did not inhibit digester performance.
Degradation of phosphonates in natural water can occur at slow to moderate rates in the presence and absence of sediments. This degradation is likely a combination of biological and physical processes, supported by the findings of Saeger et al (1978) which show that degradation is higher in the presence of light than in unlit conditions.
A microcosm study (150-175 m1 water; 20 to 60 grams sediment) conducted by Saeger V W (undated, believed to be 1979) demonstrated that microcosm variables: aeration vs nitrogen purge; light vs dark; active vs sterile, did not seem to significantly affect the rate of removal of 14C from the water column. This indicated that non-degradative mechanisms dominate removal from water column, though biodegradation under both oxygen-rich and oxygen-deficient conditions does occur at relatively slow rates.
Some studies have observed a low level of degradation in apparently sterile conditions, in dark conditions. Some authors have referred to hydrolysis taking place. This is unlikely based on structural considerations. Some other process is more likely to be taking place, e.g. oxidation or an internal reaction.
In a 12-unit study over 119 days in four soil types (Olivette Garden, Iowa Farm, St Charles Ray silt loam and Meramec river bank), using radiolabelled ATMP and 14CO2 evolution, 7.11 – 14.64% degradation occurred. 0.63 – 0.82% degradation occurred in parallel tests with soils sterilised using sodium azide (Saeger et al., Monsanto, 1977).
In a 148 day CO2 evolution test using two standard soils, St Charles Ray silt loam and Meramec river bank, in a 20 unit apparatus, 14.18% degradation was seen. In soils sterilized using sodium azide, after 148 days, 0.28% was degraded (Saeger et al., Monsanto, 1978).
Phosphonate-utilising bacteria are ubiquitous (Schowanek and Verstraete (1990), but soil biodegradation studies are limited. The slow mineralisation shown in some studies (Steber and Wierich (1986), Steber and Wierich (1987)) is probably a result of abiotic processes.
Information on Registered Substances comes from registration dossiers which have been assigned a registration number. The assignment of a registration number does however not guarantee that the information in the dossier is correct or that the dossier is compliant with Regulation (EC) No 1907/2006 (the REACH Regulation). This information has not been reviewed or verified by the Agency or any other authority. The content is subject to change without prior notice.
Reproduction or further distribution of this information may be subject to copyright protection. Use of the information without obtaining the permission from the owner(s) of the respective information might violate the rights of the owner.
