Registration Dossier
Registration Dossier
Data platform availability banner - registered substances factsheets
Please be aware that this old REACH registration data factsheet is no longer maintained; it remains frozen as of 19th May 2023.
The new ECHA CHEM database has been released by ECHA, and it now contains all REACH registration data. There are more details on the transition of ECHA's published data to ECHA CHEM here.
Diss Factsheets
Use of this information is subject to copyright laws and may require the permission of the owner of the information, as described in the ECHA Legal Notice.
EC number: 701-325-7 | CAS number: -
- Life Cycle description
- Uses advised against
- Endpoint summary
- Appearance / physical state / colour
- Melting point / freezing point
- Boiling point
- Density
- Particle size distribution (Granulometry)
- Vapour pressure
- Partition coefficient
- Water solubility
- Solubility in organic solvents / fat solubility
- Surface tension
- Flash point
- Auto flammability
- Flammability
- Explosiveness
- Oxidising properties
- Oxidation reduction potential
- Stability in organic solvents and identity of relevant degradation products
- Storage stability and reactivity towards container material
- Stability: thermal, sunlight, metals
- pH
- Dissociation constant
- Viscosity
- Additional physico-chemical information
- Additional physico-chemical properties of nanomaterials
- Nanomaterial agglomeration / aggregation
- Nanomaterial crystalline phase
- Nanomaterial crystallite and grain size
- Nanomaterial aspect ratio / shape
- Nanomaterial specific surface area
- Nanomaterial Zeta potential
- Nanomaterial surface chemistry
- Nanomaterial dustiness
- Nanomaterial porosity
- Nanomaterial pour density
- Nanomaterial photocatalytic activity
- Nanomaterial radical formation potential
- Nanomaterial catalytic activity
- Endpoint summary
- Stability
- Biodegradation
- Bioaccumulation
- Transport and distribution
- Environmental data
- Additional information on environmental fate and behaviour
- Ecotoxicological Summary
- Aquatic toxicity
- Endpoint summary
- Short-term toxicity to fish
- Long-term toxicity to fish
- Short-term toxicity to aquatic invertebrates
- Long-term toxicity to aquatic invertebrates
- Toxicity to aquatic algae and cyanobacteria
- Toxicity to aquatic plants other than algae
- Toxicity to microorganisms
- Endocrine disrupter testing in aquatic vertebrates – in vivo
- Toxicity to other aquatic organisms
- Sediment toxicity
- Terrestrial toxicity
- Biological effects monitoring
- Biotransformation and kinetics
- Additional ecotoxological information
- Toxicological Summary
- Toxicokinetics, metabolism and distribution
- Acute Toxicity
- Irritation / corrosion
- Sensitisation
- Repeated dose toxicity
- Genetic toxicity
- Carcinogenicity
- Toxicity to reproduction
- Specific investigations
- Exposure related observations in humans
- Toxic effects on livestock and pets
- Additional toxicological data
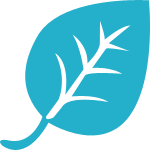
Bioaccumulation: aquatic / sediment
Administrative data
Link to relevant study record(s)
Description of key information
Bioaccumulation poses no concern.
Key value for chemical safety assessment
Additional information
- Brix K, DeForest, DK, Adams WJ (2001). Assessing acute and chronic copper risks to freshwater aquatic life using species sensitivity distributions for different taxonomic groups. Environmental Toxicology and Chemistry 20(8):1846-56.
- ICMM (2007). MERAG: Metals Environmental Risk Assessment Guidance ISBN: 978-0-9553591-2-5, 80 p
- McCance RA, Widdowson EM (1938). The absorption and excretion of iron following oral and intravenous administration. J. Phys. 94:148
- Merck (2001) The MERCK Index 13th Edition. Merck and Co Inc, Whitehouse Station, NJ.
- Neilands JB (1972). Evolution of Biological Iron Binding Centers. Struct. Bonding 11:145-70.
- WHO (1983) 571. Iron. Toxicological evaluation of certain food additives and contaminants. WHO Food Additives Series, No. 18, nos 554-573 on INCHEM http://www.inchem.org/documents/jecfa/jecmono/v18je18.htm
- Ando T, Yamamoto M, Tomiyasu T, Hashimoto J, Miura T, Nakano A, Akiba S (2002). Bioaccumulation of mercury in a vestimentiferan worm living in Kagoshima Bay, Japan. Chemosphere 49:477–84.
- ATSDR Agency for Toxic Substances and Disease Registry (2008). Draft Toxicological Profile for Manganese. U.S. Department of Health and Human Services, Public Health Service, Atlanta, Georgia, U.S.A. 539 p
- Baden SP, Eriksson SP, Weeks JM (1995). Uptake, accumulation and regulation of manganese during experimental hypoxia and normoxia by the decapod Nephrops norvegicus (L.). Marine Pollution Bulletin 31(1–3):93–102.
- Folsom TR, Young DR, Johnson JN, et al (1963). Manganese-54 and zinc-65 in coastal organisms of California. Nature 200:327-9.
- ICMM (2007). MERAG: Metals Environmental Risk Assessment Guidance ISBN: 978-0-9553591-2-5, 80 p
- Ichikawa R (1961) On the concentration factors of some important radionuclides in marine food organisms. Bulletin of the Japanese Society of Scientific Fisheries 27:66–74.
- Kwasnik GM, Vetter RJ, Atchison GJ (1978) The uptake of manganese-54 by green algae (Protococcoidal chlorella), Daphnia magna, and fathead minnows (Pimephales promelas). Hydrobiologia 59:181–185.
- Miller DW, Vetter RJ, Atchinson GJ (1980) Effect of temperature and dissolved oxygen on uptake and retention of 54Mn in fish. Health Physics 38(2):221–5.
- Rouleau C, Pelletier E, Tjälve H (1992). Bioconcentration and distribution of 54Mn2+ and effects of chelating agents in the brown trout, Salmo trutta. Canadian Technical Report of Fisheries and Aquatic Sciences 1863:330–9.
- Struck BD, Pelzer R, Ostapczuk P, Emons H, Mohl C (1997). Statistical evaluation of ecosystem properties influencing the uptake of As, Cd, Co, Cu, Hg, Mn, Ni, Pb and Zn in seaweed (Fucus vesiculosus) and common mussel (Mytilus edulis). Science of the Total Environment 207:29–42.
- Stokes PM, Campbell PGC, Schroeder WH, Trick C, France RL, Puckett KJ, LaZerte B, Speyer M, Hanna JE, Donaldson J (1988). Manganese in the Canadian environment. Ottawa, Ontario, National Research Council of Canada, Associate Committee on Scientific Criteria for Environmental Quality (NRCC No. 26193).
- Thompson SE, Burton CA, Quinn DJ, et al (1972). Concentration factors of chemical elements in edible aquatic organisms. Lawrence Livermore Laboratory, Bio-Medical Division, University of California, Livermore, CA
- WHO World Health Organization (2004 and 2005). Manganese and its Compounds: Environmental Aspects. Concise International Chemical Assessment Document 63, Corrigenda published by 12 April 2005 have been incorporated. Self-published, Geneva, Switzerland
- Herrmann J, Frick K (1995). Do Stream Invertebrates Aluminium at low pH conditions? Water, Air and Soil Pollution 85:407-12.
- Imray P, Moore MR, Callan PW, Lock W (1998). Aluminium: report of an international meeting 20-21 April 1995, Brisbane. ISBN 0 642 28157 2 ISSN 1327-4775 Published by the National Environmental Health Forum. National Environmental Health Forum Monographs Metal Series No. 1
- Vangheluwe M, Vercaigne I, Vandenbroele M, Shtiza A, Heijerick D (2010). White Paper on exposure based waiving for iron and aluminium in soil and sediments. ARCHE, Stapelplein 70, Box 104, 9000 Gent, Belgium
Generally aquatic biota regulate actively their internal concentrations of metals by active transport, storage or a combination of both. As a result of these processes which do often not discriminate non-essential metals, an inverse relationship gets established between water concentrations and the corresponding BCF of most metals. This means that organisms accumulate metals unspecifically to meet their metabolic requirements, whereat non-essential metals are moderately accumulated as well. At higher water concentrations, organisms with active regulation mechanisms are able to excrete excess metals or limit their uptake (ICMM 2007, Brix & DeForest 2000). If such mechanisms apply, the typical figures are that metal concentrations in tissue based on a range of exposure concentrations may be quite similar, but the BCFs will be quite variable, i.e., higher BCFs occur at lower exposure concentrations and lower BCFs at higher exposure concentrations (ICMM 2007). The formation of metalorganic species on the other hand would lead to figures as known from organic chemicals. In conclusion not primarily the magnitude of measured BCF (provided the value is not alarmingly high for non-essential metals) is indicative for evaluation but the establishment of an inverse relationship rather than apparent independence or a proportional one. Decreasing values for BCF in higher trophic levels are indicative for the absence of concern. The latter would be of concern while the former one is just the normal metal behaviour giving no indication for the formation of bioaccumulating species.
Iron
The read across for the key study is justified on the basis that members in the category exhibit similar values for all areas of the property data set and thus share common environmental fate and behaviour. The salts will dissociate immediately in aqueous solution under normal environmental conditions. The key study of CERI (2001) studied the bioconcentration of iron using an appropriate study guideline (OECD TGD 305). The study was conducted using ferrous sulphate and it was shown that BCF values were less than 20 for fish (Cyprinus sp) in a 28 day study using flow-through (CERI 2001). In another supporting study (Andersen 1997), a bioconcentration factor of 13.5 - 91.7% was obtained for iron uptake in the fry (post yolk absorption stage) of brown trout. The level of iron in the intact eggs showed a dose-dependent increase following treatment. The levels of the embryo in the egg membranes did not significantly differ between treated groups and controls. About 5% of the accumulated tracer was observed in the embryo after 4 days of exposure to 59Fe. Iron accumulation in the fry was suggested to increase with development of the gills. The bioconcentration factor decreases with increasing iron exposure, constituting thus a metal typical inverse relationship. Pentreath (1973) reported that the mollusc, mussel (Mytilus edulis) had highest measured bioaccumulation factors of 2756 – 9622 when exposed to initial total iron concentration of 0.009 mg/L (mean seawater concentration is 0.0034 mg/L according to Turekian KK (1968 Oceans. Prentice-Hall) for 42 days at 10 °C. The results support a sequestering mechanism for active uptake of iron from seawater into the organism, which reflects an adaptation to the generally low iron bioavailability in marine environments rather than an effect of relevance for the hazard assessment.
Biologically, iron is an essential trace element for organisms including micro-organisms, plants and animals. Iron plays an important role in biological processes, and iron homeostasis is under strict control (McCance & Widdowson 1938). Many organisms actively regulate uptake of iron into cells. It is an important factor in oxygen transport in the blood, oxidative metabolism, electron transport, nitrogen fixation and other biological processes in cells. Iron is a constituent of haemoglobin, enzyme systems and chlorophyll molecules essential to life. Iron can be bound into various chelate complexes or proteins in biological material (Merck 2001, Neilands 1972, WHO 1983).
Iron is a biologically essential metal actively taken up into organisms. The available data on iron concentrations in the environment show the way that organisms display adaptation to the high background concentrations of iron.
Iron is the forth-most abundant element in the Earth's crust (4.7% by mass) occurring naturally as almost as oxide and hydroxide. Although it is widely distributed and present in all environmental media the highest levels were in soil. Vangheluwe et al 2010 calculated the soil background concentration of iron species to be about 18.5 to 20.6 g/ kg soil d.w. Despite its presence no accumulation in wildlife biota is described in the literature. This supports the suggestion of a low potential for bioaccumulation.
Manganese
Manganese is an essential element (WHO 2004 and 2005) and is, therefore, actively assimilated and utilized by both plants and animals. It has been established that while lower organisms (e.g., plankton, aquatic plants, and some fish) can significantly bioconcentrate manganese, higher organisms (including humans) tend to maintain manganese homeostasis (EPA 1984, Folsom et al 1963, Thompson et al 1972). This indicates that the potential for biomagnification of manganese from lower trophic levels to higher ones is low, and it does not appear that additional research in this area is essential at this time (ATSDR 2008).
Table: Manganese BCFs reported in the literature
Taxon |
BCF |
Reference |
Trophic level |
Marine and freshwater plants |
10,000 - 20,000 |
Thompson et al 1972 |
Primary producers |
Marine macroalgae |
300 - 5,500 |
Folsom et al 1963 |
|
Phytoplankton |
2,500 - 6,300 |
Folsom et al 1963 |
|
Algae (Chlorella) |
911 |
Kwasnik et al 1978 |
|
Annelids |
140,000 - 300,000 |
Ando et al 2002 |
Primary consumers |
Invertebrates |
10,000 - 40,000 |
Thompson et al 1972 |
|
Intertidal mussels |
800 - 830 |
Folsom et al 1963 |
|
Daphnids (Daphnia magna) |
65 |
Kwasnik et al 1978 |
|
Costal fish |
35 - 930 |
Folsom et al 1963 |
Primary and/or Secondary consumers |
Fish |
100 - 600 |
Thompson et al 1972 |
|
Marine fish |
ca. 100 |
Ichikawa (1961) |
|
Fathead minnows (Pimephales promelas) |
23 |
Kwasnik et al 1978 |
Secondary consumers |
In general, these data indicate that lower organisms such as algae have larger BCFs than higher organisms (ATSDR 2008). In conclusion no concern for biomagnification of manganese is given as decreasing concentrations up the food-chain occurs. Nonetheless Stokes et al (1988) state weak biomagnification is questionable if the media levels were comparable. This is crucial as the decreasing media concentration increases the BCF.
Table: Determinants and their qualitative influence on manganese BCF
Decreasing determinant value |
Effect with regard to BCF |
Reference |
Temperature |
Decrease |
Miller et al 1980 |
Dissolved oxygen |
None |
Miller et al 1980, |
pH |
Increase |
Rouleau et al 1996 |
Salinity |
Struck et al 1997 |
|
Media concentration |
ICMM (2007). |
Due to the fact that Kwasnik et al (1978) studied the BCF with comparable media concentrations in three trophic levels using organisms, which are standard representatives for regulatory purposes, the figures of this publication are considered valid and conclusive. It is assessed that enrichment of manganese in organisms poses no concern.
Aluminium
Aluminium is the third-most abundant element in the Earth's crust (7.57% by mass). Therefore natural sources of aluminium far outweigh those produced via anthropogenic sources. In fact, all of the anthropogenic activities releasing aluminium were identified as minor sources (Imray et al 1998). Aluminium represents the most frequent metal occurring naturally almost as oxide and hydroxide. Although it is widely distributed and present in all environmental media the highest levels were in soil. Vangheluwe et al 2010 calculated the soil background concentration of aluminium species to be about 27.8 to 29.9 g/ kg soil d.w. Despite its presence no accumulation in wildlife biota is described in the literature. This supports the suggestion of a low potential for bioaccumulation (Imray et al 1998).
Despite the major sources of aluminium are natural the species are not in a bioavailable, dissolved form but in a particulate form. The effects were thus triggered by processes effecting this mobilisation. As an amphoteric element the aluminium is highly pH dependant, increasing at both low and high pH values. This fact, together with the large reservoir of aluminium in soils and sediments, means that aluminium concentrations can be much more significantly influenced by changes in pH rather than by environmental release. This applies particularly to acidic or poorly buffered media (Imray et al 1998). Natural sources include the breakdown of iron pyrites (iron sulphide), by bacteria, forming sulphuric acid during the dry season which is subsequently released at the start of the wet season (first flush) and acid precipitation caused by the release of volatile organic compounds from plants, such as eucalypts, forming formic acid. Anthropogenic sources include direct sources, such as acid precipitation caused by chemical releases from transport (NOx), industry (SO2), animal husbandry (HNO3) and indirect sources of acidity, such as: the first melt-water of spring (which has accumulated sulphuric acid), contaminated land and agricultural chemical applications. In consequence the buffering capacity of the local soil type and underlying geology will determine if the effects are actually manifested (Imray et al 1998).
The available evidence shows the absence of aluminium biomagnification across trophic levels both in the aquatic and terrestrial food chains. The existing information suggests not only that aluminium does not biomagnify, but rather that it tends to exhibit biodilution at higher trophic levels in the food chain. More detailed information can be found in the attached document (Arche White paper on waiving for secondary poisoning for Al and Fe compounds final report 25-01-2010.pdf). BCFs for aluminium can be found to range from quite low (~100) to quite high values 11,000. This variance can in large part be explained by the difference in exposure conditions for the various studies. The inverse relationship between water and BCF/BAF values limits the ability to describe hazard as a result of the size of the BCF, i.e. the most pristine ecosystems have the highest BCFs. A better approach is to directly assess the concentrations of Al at various trophic levels in the ecosystem.
Herrmann and Frick (1995) studied the accumulation of aluminium at low pH conditions in benthic invertebrates with time and representing different functional feeding groups (predators and detritus feeders). Invertebrates of different taxa and feeding type were collected in springtime, when acidity and A1 levels mostly increase from seven streams in southern Sweden. Four of the streams typically had pH values of 4 - 4.5 and contained 0.40 - 0.70 mg inorganic A1/L. The other three streams showed pH values around 6 and A1 concentrations of 0.05 mg inorganic A1/l. For most taxa that could be compared, the animals from the most acidic streams (pH 4) contained more A1 than those from the less acid streams (pH 6). At both pH levels there was a clear tendency that predators contained significantly less amounts of aluminium than shredders. The latter results do not support the hypothesis that aluminium can be accumulated along a food chain in an acidic environment.
Information on Registered Substances comes from registration dossiers which have been assigned a registration number. The assignment of a registration number does however not guarantee that the information in the dossier is correct or that the dossier is compliant with Regulation (EC) No 1907/2006 (the REACH Regulation). This information has not been reviewed or verified by the Agency or any other authority. The content is subject to change without prior notice.
Reproduction or further distribution of this information may be subject to copyright protection. Use of the information without obtaining the permission from the owner(s) of the respective information might violate the rights of the owner.
