Registration Dossier
Registration Dossier
Data platform availability banner - registered substances factsheets
Please be aware that this old REACH registration data factsheet is no longer maintained; it remains frozen as of 19th May 2023.
The new ECHA CHEM database has been released by ECHA, and it now contains all REACH registration data. There are more details on the transition of ECHA's published data to ECHA CHEM here.
Diss Factsheets
Use of this information is subject to copyright laws and may require the permission of the owner of the information, as described in the ECHA Legal Notice.
EC number: 701-246-8 | CAS number: -
- Life Cycle description
- Uses advised against
- Endpoint summary
- Appearance / physical state / colour
- Melting point / freezing point
- Boiling point
- Density
- Particle size distribution (Granulometry)
- Vapour pressure
- Partition coefficient
- Water solubility
- Solubility in organic solvents / fat solubility
- Surface tension
- Flash point
- Auto flammability
- Flammability
- Explosiveness
- Oxidising properties
- Oxidation reduction potential
- Stability in organic solvents and identity of relevant degradation products
- Storage stability and reactivity towards container material
- Stability: thermal, sunlight, metals
- pH
- Dissociation constant
- Viscosity
- Additional physico-chemical information
- Additional physico-chemical properties of nanomaterials
- Nanomaterial agglomeration / aggregation
- Nanomaterial crystalline phase
- Nanomaterial crystallite and grain size
- Nanomaterial aspect ratio / shape
- Nanomaterial specific surface area
- Nanomaterial Zeta potential
- Nanomaterial surface chemistry
- Nanomaterial dustiness
- Nanomaterial porosity
- Nanomaterial pour density
- Nanomaterial photocatalytic activity
- Nanomaterial radical formation potential
- Nanomaterial catalytic activity
- Endpoint summary
- Stability
- Biodegradation
- Bioaccumulation
- Transport and distribution
- Environmental data
- Additional information on environmental fate and behaviour
- Ecotoxicological Summary
- Aquatic toxicity
- Endpoint summary
- Short-term toxicity to fish
- Long-term toxicity to fish
- Short-term toxicity to aquatic invertebrates
- Long-term toxicity to aquatic invertebrates
- Toxicity to aquatic algae and cyanobacteria
- Toxicity to aquatic plants other than algae
- Toxicity to microorganisms
- Endocrine disrupter testing in aquatic vertebrates – in vivo
- Toxicity to other aquatic organisms
- Sediment toxicity
- Terrestrial toxicity
- Biological effects monitoring
- Biotransformation and kinetics
- Additional ecotoxological information
- Toxicological Summary
- Toxicokinetics, metabolism and distribution
- Acute Toxicity
- Irritation / corrosion
- Sensitisation
- Repeated dose toxicity
- Genetic toxicity
- Carcinogenicity
- Toxicity to reproduction
- Specific investigations
- Exposure related observations in humans
- Toxic effects on livestock and pets
- Additional toxicological data
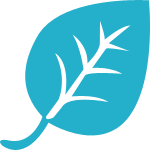
Biodegradation in water and sediment: simulation tests
Administrative data
- Endpoint:
- biodegradation in water and sediment: simulation testing, other
- Type of information:
- calculation (if not (Q)SAR)
- Adequacy of study:
- supporting study
- Reliability:
- 2 (reliable with restrictions)
- Rationale for reliability incl. deficiencies:
- study well documented, meets generally accepted scientific principles, acceptable for assessment
- Justification for type of information:
- The substance ‘Oligomerisation products of beta-pinene’ (EC number: 701-246-8) received a final decision from ECHA with regards to REACH (EC 1907/2006) in 2020. In this decision ECHA requested identification of degradation products without specifically mandating a test. If this was a simple well-defined substance, a simulation test would be an obvious choice, but the nature of the test substance means this would be extremely challenging. However, without a mandated test, we are left with the situation where we have to do the best we can to meet the final decision whilst being unaware of how high the barrier to acceptance will be.
More details on the tests and investigations made to satisfy this requirement are given in the attached document.
Data source
Reference
- Reference Type:
- study report
- Title:
- Unnamed
- Year:
- 2 021
- Report date:
- 2021
Materials and methods
Test guideline
- Qualifier:
- no guideline available
- Principles of method if other than guideline:
- Degradation products require further consideration if they are detected in experimental studies, with specific triggers at >10% at any time during the study or if a degradation product concentration is continuously increasing or appears stable during the study (ECHA, 2017). If it is unfeasible to determine degradation product level experimentally other methods may be used as a proxy to predict the relative concentrations of parent/degradation product. RIVM (2008) described a framework for the assessment of degradation products that included a survey of predicted or measured degradation product level that was based on approaches used by Health Canada. In this framework stable degradation products are first identified or predicted and the relative quantity vs. parent compound is determined. It is proposed here that a >10% threshold is used, in line with REACH PBT guidance, to screen out minor degradation products that may be highlighted as a possible persistence concern. Further screening will be undertaken to identify degradation products that are continuously increasing or stable.
In order to determine the likely formation and relative persistence of degradation products formed from beta-pinene oligomers the following steps were undertaken:
• Selection of one or two representative beta-pinene dimer structures based on recognised grouping approaches.
• Prediction of pinene dimer degradation products using prediction models.
• Prediction relative biodegradability of degradation products compared to parent chemical and estimation of degradation rates.
• Input degradation kinetics into first order biodegradation model to inform likelihood of any degradation product triggering the 10% concentration threshold based on REACH rule.
Pathway prediction was principally performed using enviPath (www.enviPath.org) which is a successor of the University of Minnesota Biocatalysis/Biodegradation Database and Pathway Prediction System (UM-BBD/PPS). EnviPath predicts microbial biotransformation reactions using a substructure search, a rule base and atom-to-atom mapping. The system is able to recognize organic functional groups in a substance and predict transformations through generalized biotransformation rules. The biotransformation rules are based on reactions in the enviPath database and/or in the scientific literature.
Primary biodegradation half-lives (HL) of degradation products predicted through enviPath or other approaches. Briefly, The estimated environmental half-lives were evaluated by comparison empirical data to model estimates from BIOWIN biodegradation models 1, 3, 4 and 5. HL is determined by running all four models (BIOWIN 1, 3, 4, and 5) and averaging values.
The relative concentration of predicted degradation products was determined using a pathway modeller in Excel. This graphical visualiser allows identification of predicted degradation products that form at >10% of parent chemical. Degradation products are tracked sequentially, assuming 1st order kinetics, and the degradation of each degradation product is shown over time alongside the degradation of the parent chemical. The calculation of each degradation product in a predicted pathway is determined by calculating the balance of degradation product formation and degradation product degradation. In pathways where branching occurs (i.e. where multiple degradation products are predicted from the same previous structure), the proportion of mass transferred to each degradation product is based on the relative probability value determined by enviPath. - GLP compliance:
- no
Test material
- Reference substance name:
- Oligomerisation products of beta-pinene
- EC Number:
- 701-246-8
- Molecular formula:
- Variable (dimer = C20-H34)
- IUPAC Name:
- Oligomerisation products of beta-pinene
- Test material form:
- liquid
Constituent 1
- Radiolabelling:
- no
- Remarks:
- Not possible to radio-label natural origin UVCB material
Results and discussion
- Transformation products:
- yes
Identity of transformation productsopen allclose all
- No.:
- #1
Reference
- Reference substance name:
- Unnamed
- IUPAC name:
- [4-[1,3-dimethyl-3-(4-methylcyclohex-3-en-1-yl)butyl]cyclohexen-1-yl]methanol
- Molecular formula:
- C20H34O
- Molecular weight:
- 290.48
- SMILES notation:
- CC1=CCC(CC1)C(C)(C)CC(C)C2CC=C(CC2)CO
- No.:
- #2
Reference
- Reference substance name:
- Unnamed
- IUPAC name:
- [4-[1,1-dimethyl-3-(4-methylcyclohex-3-en-1-yl)butyl]cyclohexen-1-yl]methanol
- Molecular formula:
- C20H34O
- Molecular weight:
- 290.48
- SMILES notation:
- CC1=CCC(CC1)C(C)CC(C)(C)C2CC=C(CC2)CO
- No.:
- #3
Reference
- Reference substance name:
- Unnamed
- IUPAC name:
- 2-methyl-2,4-bis(4-methylcyclohex-3-en-1-yl)pentan-1-ol
- Molecular formula:
- C20H34O
- Molecular weight:
- 290.48
- SMILES notation:
- 2-methyl-2,4-bis(4-methylcyclohex-3-en-1-yl)pentan-1-ol
- No.:
- #4
Reference
- Reference substance name:
- Unnamed
- IUPAC name:
- 4-[2-(4-isopropylcyclohexen-1-yl)-1,1-dimethyl-ethyl]cyclohexene-1-carbaldehyde
- Molecular formula:
- C20H32O
- Molecular weight:
- 288.48
- SMILES notation:
- CC(C)C1CC=C(CC1)CC(C)(C)C2CC=C(CC2)C=O
- No.:
- #5
Reference
- Reference substance name:
- Unnamed
- IUPAC name:
- 3-(4-isopropylcyclohexen-1-yl)-2-methyl-2-(4-methylcyclohex-3-en-1-yl)propan-1-ol
- Molecular formula:
- C20H34O
- Molecular weight:
- 290.48
- SMILES notation:
- CC(C)C1CC=C(CC1)CC(C)(CO)C2CC=C(C)CC2
- No.:
- #6
Reference
- Reference substance name:
- Unnamed
- IUPAC name:
- 3-(4-isopropylcyclohexen-1-yl)-2-methyl-2-(4-methylcyclohex-3-en-1-yl)propanal
- Molecular formula:
- C20H32O
- Molecular weight:
- 288.47
- SMILES notation:
- CC(C)C1CC=C(CC1)CC(C)(C=O)C2CC=C(C)CC2
- No.:
- #7
Reference
- Reference substance name:
- Unnamed
- IUPAC name:
- 2-[3-(4-carboxylatocyclohex-3-en-1-yl)-1,1-dimethyl-butyl]hexanedioate
- Molecular formula:
- C19H27O6
- Molecular weight:
- 351.41
- SMILES notation:
- CC(CC(C)(C)C(CCCC(=O)[O-])C(=O)[O-])C1CCC(=CC1)C(=O)[O-]
- No.:
- #8
Reference
- Reference substance name:
- Unnamed
- IUPAC name:
- 4-(3,4-dihydroxy-4-methyl-cyclohexyl)-2-methyl-2-(4-methylcyclohex-3-en-1-yl)pentanoate
- Molecular formula:
- C20H33O4
- Molecular weight:
- 337.47
- SMILES notation:
- CC(CC(C)(C1CCC(=CC1)C)C(=O)[O-])C2CCC(C)(O)C(O)C2
- Details on transformation products:
- Full details on the assessment of degradation products and results can be found below in "Any other information on results"
Transformation products
#1 relates to Clade 1 of dimer #1 (Table 3)
#2 relates to Clade 2 of dimer #1 (Table 3)
#3 relates to Clade 3 of dimer #1 (Table 3)
#4-6 relate to dimer #2 (Table 3)
*The following structures did not achieve the threshold for degradation products (formation at 10% of parent molecule), but are mentioned as premature termination steps in the modelling pathway. They would be expected to undergo further degradation.*
#7 relates to Clades 1 & 2 of dimer 1 (Table 3)
#8 relates to Clade 3 of dimer 1 (Table 3)
Any other information on results incl. tables
Selection of Representative Structures
Full compositional details of oligomerisation products of beta-pinene, a UVCB made from natural origin materials, were not determinable and are unlikely to be fully elucidated (analytical characterisation is given in Section 1.4 of IUCLID). As a UVCB the substance is composed of multiple constituents, the type and proportions of which are determined by the feedstock and the process used to manufacture the substance. The pinene-fraction feedstock for the polymerisation process is of natural origin, is expected to show variability in isomeric form of pinene; further details of manufacturing process and potential isomers of feedstock are given in Sections 1.2 and 1.4 of IUCLID. The structures and relative amounts of isomers present prior to dimerization are important when considering the final product. The variety of isomers produced during the initial isomerisation process leads to an extremely complex distribution of constituents. These are likely to include oligomers with homologous and heterogeneous constituents and different conjugation locations.
Considering the absence of specific information regarding the composition of dimers and higher oligomers an effort was made to search the research literature to identify relevant structures to be used as a basis for degradation product prediction (Table 3 - attached picture below). The search identified 10 different dimers which were predominantly linear but also included several fused bicylic structures. This collection of 10 structures also included the dimer originally provided by the Sponsor. In total, three common descriptors were identified in the structures presented in table 3 that make it possible to describe dimers generally:
- Linear monomers linked via alkyl conjugation with a quaternary carbon and five methyl alkyl groups (structures 1 - 4, Table 3).
- Linear monomers linked via alkyl conjugation with two quaternary carbons and five methyl groups (structures 5 – 8, Table 3).
- Fused bicylic and five methyl groups (structures 9 – 10, Table 3).
No specific trimer and tetramer structures were identified during the literature search. As such generic configurations, based on repeating limonene units, were considered representative structures for these oligomers (Table 3).
Degradation Product Predictions
Degradation product and pathway predictions of the pinene oligomers shown in Table 3 were predicted using enviPath (Probability threshold 0.5). As these dimers are hydrocarbons, biodegradation likely proceeds via β-oxidation or a combination of α and β-oxidation. The mineralization of hydrocarbons requires the introduction of functional groups to access β-oxidation to yield central degradation products, e.g. acetyl-CoA. In many aerobic microorganisms molecular oxygen serves as reactive agent to functionalize monoterpenes (Marmulla and Harder, 2014). For cyclic hydrocarbons, β-oxidation proceeds after ring opening and the functionalisation of the structure to a carboxylic acid.
Dimer Structure 1 (Table 3)
Dimer 1 (Table 3), is structurally similar to limonene, but also includes a quaternary carbon. General wisdom holds that increased branching causes increased resistance to biodegradation. Resistance becomes exceptionally great when quaternary branching occurs. Therefore, conceptually we might expect the biodegradation of dimer 1 to begin with functionalisation via oxygen, proceed through ring opening and β-oxidation and finally the removal of the more difficult to degrade quaternary carbon. In total more than 150 degradation products were identified by enviPath for dimer 1 in a multigeneration prediction. Progression of biodegradation and shows three major branches (described as Clades 1, 2 & 3) of degradation products produced because of a split at the first step in the biodegradation process. The three-way split represents a predicted functionalisation (via oxygenation) at different points on the dimer. Clades 1 and 2 represent degradation products derived from an initial hydroxylation of alternate cyclic rings, whereas clade 3 degradation products are produced from an initial hydroxylation of a methyl group branch from the central quaternary carbon.
In clades 1 and 2, both initially functionalised on the cyclic ring of the dimer, biodegradation is predicted to proceed via ring opening and β-oxidation. In addition, the opposite ring is carboxylated and thus susceptible to opening and further β-oxidation. As anticipated the quaternary carbon remained present. In clade 3, degradation products resulted in a carboxylated quaternary carbon branch and an oxygenated cyclic ring. In fact, predictions in all clades end with degradation products that functionally (at least) appear susceptible to further biodegradation. The reason behind these ‘premature’ terminations in degradation product predictions is likely due to the absence of an applicable relative reasoning rule for the parent degradation product (Fenner et al., 2008). Subsequent analysis of ‘terminal’ degradation products by UM-PPS, where relative reasoning is not used and predictions are based on transformation rules and the presence of functional groups, indicate continued transformation and yielding of degradation products used in central metabolism. Structures representative of the premature termination steps are given as #7 (Clades 1 & 2) and #8 (Clade 3) in the table of transformation products above. These two structures did not achieve the threshold for degradation products (formation at 10% of parent molecule) and are expected to degrade further.
Dimer structures 2 -10 (Table 3)
The remaining nine pinene dimers were processed as described in Table 3 above. Of these only four (2, 6, 9 and 10) were predicted to generate transformations products using enviPath and only one of these (dimer 2) produced degradation products above the 10% threshold.
For dimers that did not produce any predicted transformation pathways using enviPath, a separate manual assessment was performed. The use of UM-PPS was discounted because the number of predicted degradation products would be too difficult to process. For example, predictions by UM-PPS of Dimer 8 produce 47 possible degradation products as a first transformation step. To limit this combinatorial explosion BioTransformer (http://biotransformer.ca) was used to predict three steps of transformation for dimers 3, 4, 5, 7 and 8 (Table 3). BioTransformer is an open access software tool that includes a Metabolism Prediction Tool. It combines a knowledge-based, and a machine learning-based approach to predict the metabolism of small molecules including environmental microbial transformations. A comprehensive evaluation of BioTransformer showed it was able reproduce 100% of the transformations and degradation products predicted by the EAWAG Pathway Prediction system whilst using relative reasoning to identify the most probable transformations (Djoumbou-Feunang et al., 2019). A summary of the number of degradation products predicted by BioTransformer for dimers 3, 4, 5, 7 and 8 are provided in Table 5.
Table 5. Summary of degradation products predicted from various Pinene dimers by BioTransformer |
|||
|
Transformation Step 1 |
Transformation Step 2 |
Transformation Step 3 |
Dimer 3 |
7 |
26 |
78 |
Dimer 4 |
7 |
30 |
92 |
Dimer 5 |
4 |
10 |
23 |
Dimer 7 |
4 |
12 |
28 |
Dimer 8 |
5 |
19 |
57 |
Biodegradability Prediction
Dimer structure 1 (Table 3)
In a next step the degradation product structures predicted by EnviPath were run through the BIOWIN biodegradation QSAR models (Boethling and Costanza, 2010) and HLs calculated as described by Arnot et al., (2005). A small number of degradation products, approximately 10%, were estimated to be more persistent than the parent dimer. However, these degradation products were never more than 50% persistent than the parent dimer and never achieved the threshold for degradation products (formation at 10% of parent molecule). In general, it was observed that persistence decreased as transformation (and oxygenation) increased.
Dimer structures 2 -10 (Table 3)
Dimer 2 was similar to Dimer 1 (described above), with only degradation product estimated to be more persistent than the parent dimer; again this degradation product did not achieve the threshold for degradation products (formation at 10% of parent molecule). For Dimers 6, 9 & 10 no degradation products were estimated to be more persistent than the parent molecule.
The remaining dimers were those whose degradation products were estimated by Biotransformer. Biotransformer predicts increasing numbers of degradation products after each transformation step and is thus likely to be susceptible to ‘combinatorial explosion’. As a consequence, the overall sensitivity and accuracy of these predictions will be reduced and the identification of most plausible degradation products difficult to determine without thorough manual checking. Considering this, it is difficult to conclude on the relative persistence of degradation products for dimers 3, 4, 5, 7 and 8.
Prediction of Degradation Product Formation above 10% Threshold
Dimer structure 1 (Table 3)
The relative proportions of the predicted degradation products were modelled using the pathway structure predicted by enviPath and their corresponding estimated HLs. As the first transformation step predicted three possible degradation products, three separate transformation pathways were modelled, with each receiving a third of the original mass of the parent. In all three clades, only the first degradation product of the pathway breached the 10% threshold - these are given as transformation products #1 (clade 1), #2 (clade 2) and # 3 (clade 3). Subsequent transformations and degradation products were consistently below the 10% threshold. It should be noted that the presented transformation pathways and degradation product dynamics of clades 1 and 2 are truncated because further branching in these pathways means it is mathematically impossible for subsequent degradation products to breach the 10% threshold.
Dimer structures 2 -10 (Table 3)
Only dimer structure 2 produced metabolites over the 10% threshold, and these are given as transformation products #4, #5 & #6.
Boethling, R. S. and Costanza, J. (2010) 'Domain of EPI suite biotransformation models', SAR QSAR Environ Res, 21(5-6), pp. 415-43
Djoumbou-Feunang, Y., Fiamoncini, J., Gil-de-la-Fuente, A., Greiner, R., Manach, C. and Wishart, D. S. (2019) 'BioTransformer: a comprehensive computational tool for small molecule metabolism prediction and metabolite identification', Journal of Cheminformatics, 11(1), pp. 2.
Fenner, K., Gao, J., Kramer, S., Ellis, L. and Wackett, L. (2008) 'Data-driven extraction of relative reasoning rules to limit combinatorial explosion in biodegradation pathway prediction', Bioinformatics, 24(18), pp. 2079-2085.
Marmulla, R. and Harder, J. (2014) 'Microbial monoterpene transformations—a review', Frontiers in Microbiology, 5, pp. 346-356.
Applicant's summary and conclusion
- Conclusions:
- Relevant degradation products of the substance have been predicted using appropriate modelling as experimental testing was not considered feasible owing to the complexity of the substance.
Information on Registered Substances comes from registration dossiers which have been assigned a registration number. The assignment of a registration number does however not guarantee that the information in the dossier is correct or that the dossier is compliant with Regulation (EC) No 1907/2006 (the REACH Regulation). This information has not been reviewed or verified by the Agency or any other authority. The content is subject to change without prior notice.
Reproduction or further distribution of this information may be subject to copyright protection. Use of the information without obtaining the permission from the owner(s) of the respective information might violate the rights of the owner.
