Registration Dossier
Registration Dossier
Data platform availability banner - registered substances factsheets
Please be aware that this old REACH registration data factsheet is no longer maintained; it remains frozen as of 19th May 2023.
The new ECHA CHEM database has been released by ECHA, and it now contains all REACH registration data. There are more details on the transition of ECHA's published data to ECHA CHEM here.
Diss Factsheets
Use of this information is subject to copyright laws and may require the permission of the owner of the information, as described in the ECHA Legal Notice.
EC number: 230-386-8 | CAS number: 7085-19-0
- Life Cycle description
- Uses advised against
- Endpoint summary
- Appearance / physical state / colour
- Melting point / freezing point
- Boiling point
- Density
- Particle size distribution (Granulometry)
- Vapour pressure
- Partition coefficient
- Water solubility
- Solubility in organic solvents / fat solubility
- Surface tension
- Flash point
- Auto flammability
- Flammability
- Explosiveness
- Oxidising properties
- Oxidation reduction potential
- Stability in organic solvents and identity of relevant degradation products
- Storage stability and reactivity towards container material
- Stability: thermal, sunlight, metals
- pH
- Dissociation constant
- Viscosity
- Additional physico-chemical information
- Additional physico-chemical properties of nanomaterials
- Nanomaterial agglomeration / aggregation
- Nanomaterial crystalline phase
- Nanomaterial crystallite and grain size
- Nanomaterial aspect ratio / shape
- Nanomaterial specific surface area
- Nanomaterial Zeta potential
- Nanomaterial surface chemistry
- Nanomaterial dustiness
- Nanomaterial porosity
- Nanomaterial pour density
- Nanomaterial photocatalytic activity
- Nanomaterial radical formation potential
- Nanomaterial catalytic activity
- Endpoint summary
- Stability
- Biodegradation
- Bioaccumulation
- Transport and distribution
- Environmental data
- Additional information on environmental fate and behaviour
- Ecotoxicological Summary
- Aquatic toxicity
- Endpoint summary
- Short-term toxicity to fish
- Long-term toxicity to fish
- Short-term toxicity to aquatic invertebrates
- Long-term toxicity to aquatic invertebrates
- Toxicity to aquatic algae and cyanobacteria
- Toxicity to aquatic plants other than algae
- Toxicity to microorganisms
- Endocrine disrupter testing in aquatic vertebrates – in vivo
- Toxicity to other aquatic organisms
- Sediment toxicity
- Terrestrial toxicity
- Biological effects monitoring
- Biotransformation and kinetics
- Additional ecotoxological information
- Toxicological Summary
- Toxicokinetics, metabolism and distribution
- Acute Toxicity
- Irritation / corrosion
- Sensitisation
- Repeated dose toxicity
- Genetic toxicity
- Carcinogenicity
- Toxicity to reproduction
- Specific investigations
- Exposure related observations in humans
- Toxic effects on livestock and pets
- Additional toxicological data
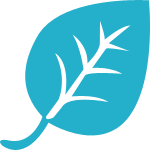
Biodegradation in water and sediment: simulation tests
Administrative data
Link to relevant study record(s)
- Endpoint:
- biodegradation in water: sediment simulation testing
- Type of information:
- experimental study
- Adequacy of study:
- supporting study
- Reliability:
- 2 (reliable with restrictions)
- Rationale for reliability incl. deficiencies:
- study well documented, meets generally accepted scientific principles, acceptable for assessment
- Qualifier:
- no guideline followed
- Principles of method if other than guideline:
- Test material degradation in different redox environments was tested within a Lincolnshire Limestone aquifer. The objective of the study was to assess whether changes in the enantiomeric fraction can be used to indicate natural attenuation in the field. The study involved sampling groundwater and relating systematic changes in enantiomeric fraction to different redox environments down gradient of the landfill. Laboratory microcosms were set up with groundwater and limestone acclimatised with the in situ microbial consortium to confirm the behaviour of the test material enantiomers under controlled conditions.
- GLP compliance:
- not specified
- Specific details on test material used for the study:
- The study concerned test material (racemic mixture of R- and S-isomers), originally deposited in a landfill.
- Radiolabelling:
- no
- Oxygen conditions:
- aerobic/anaerobic
- Inoculum or test system:
- other: in situ microbial consortium
- Details on source and properties of surface water:
- Groundwater samples were obtained from boreholes down gradient of the Ailsworth Road Landfill. It was not possible to obtain samples from within this landfill but heavily contaminated samples were collected from boreholes 28 and 29 adjacent to the Ben Johnson's Landfill site. All boreholes were already constructed with relatively long screen intervals, and were sampled after purging three times the borehole volume using a stainless steel Grundfos SPI submersible pump with PTFE tubing. Field measurements included pH, temperature, redox potential (Pt electrode) and dissolved oxygen (DO2 electrode) using a flow cell. Samples for organic analysis were collected by completely filling a 2.5 L amber glass Winchester sample bottle containing 2.5 g sodium azide (NaN3) as a preservative (biocide). The samples were analysed for major and trace inorganic species, for total organic carbon (TOC) and for test material. Further analytical details are found in Williams et al. (2001).
On flowing eastwards, the aerobic groundwater evolves naturally to become sulphate reducing and saline. Samples were categorised into redox zones.
Methanogenic/sulphatereducing conditions (boreholes 28 and 29) are identified by high TOC and the presence of methane that was actively degassing from these boreholes. Fe-reducing conditions are represented by the presence of reduced iron and low nitrate (borehole 25); nitrate reducing by low reduced iron and the presence of nitrate (boreholes 24, 4 and 32); aerobic/sub-oxic by low TOC without nitrate or reduced iron (boreholes 9, 11 and 18). Boreholes 16 and 17 and other boreholes further east in the confined zone represent the transition to sulphate-reducing/saline conditions. Since redox is known to control the fate of the test material, these redox environments formed the basis against which to assess changes in the enantiomeric fraction.
The heavily contaminated boreholes 28 and 29 down gradient from Ben Johnson's Landfill were degassing methane and contained racemic test material. - Details on source and properties of sediment:
- Crushed Lincolnshire Limestone
- Details on inoculum:
- The Lincolnshire Limestone had been suspended in boreholes for several months to allow microbial colonisation.
- Parameter followed for biodegradation estimation:
- test mat. analysis
- Details on study design:
- BACKGROUND
During the 1980s, an estimated 40 tonnes of the test material contained in tank washings was discharged into two of the sites giving concentrations of up to 39 mg/L in the waste. Subsequently, up to 8 µg/L test material was detected in a public supply borehole at Etton 2.5 km east of the landfills. Groundwater is known to flow downdip to the east of the fault where the aquifer is confined by relatively impermeable strata and where boreholes are artesian and gradient of the landfill. Laboratory microcosms were set up with groundwater and limestone acclimatised with the in situ microbial consortium to confirm the behaviour of the test material enantiomers.
MICROCOSM DESIGN
Laboratory microcosms were set up using groundwater and crushed Lincolnshire Limestone, which had been suspended in boreholes for several months to allow microbial colonisation. Each microcosm was composed of 200 mL groundwater and 50 g limestone in a 250-mL amber glass bottle. Where necessary, the groundwater was amended with racemic test material to ensure sufficiency of each enantiomer at the start of the experiment. The microcosms were incubated at 10 °C aerobically (periodically exposed to the atmosphere) and anaerobically (under a nitrogen atmosphere) and compared with identical controls sterilised with NaN3.
Four microcosms were set up with groundwater from different redox environments along the flow path. These represent methanogenic/sulphate-reducing, iron-reducing, nitrate-reducing and aerobic conditions. - Transformation products:
- yes
- Remarks:
- R-isomer degradation product in Fe/nitrate-reducing zones
- No.:
- #1
- Details on results:
- MICROCOSMS
Groundwater from Borehole 32 which lies on the boundary between aerobic and nitratereducing conditions was used in two sets of microcosms which were incubated aerobically and anaerobically.
- Borehole 32 (aerobic)
Both enantiomers disappeared over a period of 6 days in the nonsterile microcosm, while there was no change in the sterile control. The S-isomer degraded with zero-order kinetics at a rate of 1.90 mg/L/day while the R-isomer degraded more slowly at 1.32 mg/L/day.
- Borehole 32 (nitrate-reducing)
In contrast, under anaerobic conditions and with exactly the same starting material, the S-isomer did not degrade, but the R-isomer degraded with first-order kinetic rate of 0.65 mg/L/day. At the same time, there was a stoichiometric build-up of 4-chloro-2-methylphenol, which only began to degrade after the R-isomer had disappeared. Brief detection of NO2 during the experiment suggested that nitrate may have been acting as the terminal electron acceptor, but nitrate was not routinely measured. The sterile control did not change.
- Borehole 25 (Fe-reducing)
No degradation was observed in this microcosm, which initially contained only 3.85 mg/L nitrate but 15 mg/L of reduced iron. After 119 days, nitrate was added to produce a concentration of 491 mg/L nitrate, and after a lag period of 21 days the R-isomer degraded with the build-up of 4-chloro-2-methylphenol as in the anaerobic microcosm borehole 32. It is not known whether degradation would have eventually taken place in this microcosm without the addition of nitrate, but the relatively quick response suggests that nitrate was probably acting as an electron acceptor.
- Borehole 28 (methanogenic/sulphate reducing)
No change was observed in either the sterile or non-sterile microcosms using water from the heavily contaminated aquifer where the presence of sulphide and methane was indicative of methanogenic/sulphate-reducing conditions. - Validity criteria fulfilled:
- not applicable
- Conclusions:
- This study has shown that systematic change in the enantiomeric fraction of racemic test material deposited in the landfills at Helpston is a useful indicator of in situ processes and provides insight into the relative behaviour of the enantiomers under different redox conditions. Test material behaviour inferred from field data is supported by the results of microcosm experiments conducted under aerobic and anaerobic conditions. No degradation occurred in methanogenic, sulphate-reducing or iron-reducing microcosms. In nitratereducing microcosms, S-isomer did not degrade, but R-isomer degraded with zero-order kinetics at 0.65 mg/L/day. The associated buildup of 4-chloro-2-methylphenol indicated cleavage of the propionic moiety at the ether linkage, and this metabolite only degraded after the R-isomer had disappeared. In aerobic microcosms, the S-isomer degraded faster than the R-isomer both following zero-order kinetics 1.32 and 1.9 mg/L/day. The nature of the microbial community responsible for test material degradation is currently under study.
- Executive summary:
Test material degradation in different redox environments was tested within a Lincolnshire Limestone aquifer. The objective of the study was to assess whether changes in the enantiomeric fraction can be used to indicate natural attenuation in the field. The study involved sampling groundwater and relating systematic changes in enantiomeric fraction to different redox environments down gradient of the landfill. Laboratory microcosms were set up with groundwater and limestone acclimatised with the in situ microbial consortium to confirm the behaviour of the test material enantiomers under controlled conditions.
This study has shown that systematic change in the enantiomeric fraction of racemic test material deposited in the landfills at Helpston is a useful indicator of in situ processes and provides insight into the relative behaviour of the enantiomers under different redox conditions. Test material behaviour inferred from field data is supported by the results of microcosm experiments conducted under aerobic and anaerobic conditions. No degradation occurred in methanogenic, sulphate-reducing or iron-reducing microcosms. In nitratereducing microcosms, S-isomer did not degrade, but R-isomer degraded with zero-order kinetics at 0.65 mg/L/day. The associated buildup of 4-chloro-2-methylphenol indicated cleavage of the propionic moiety at the ether linkage, and this metabolite only degraded after the R-isomer had disappeared. In aerobic microcosms, the S-isomer degraded faster than the R-isomer both following zero-order kinetics 1.32 and 1.9 mg/L/day. The nature of the microbial community responsible for test material degradation is currently under study.
- Endpoint:
- biodegradation in water: simulation testing on ultimate degradation in surface water
- Type of information:
- read-across from supporting substance (structural analogue or surrogate)
- Adequacy of study:
- key study
- Justification for type of information:
- Please see the read-across justification report in Section 13 of the dossier.
- Reason / purpose for cross-reference:
- read-across source
- Compartment:
- natural water: freshwater
- % Recovery:
- 97
- Remarks on result:
- other: 10 µg/L test material
- Compartment:
- natural water: freshwater
- % Recovery:
- 100
- Remarks on result:
- other: 100 µg/L test material
- Remarks on result:
- not measured/tested
- Remarks:
- The mineralisation rate was negligible. Only amounts <5 % AR were detected as CO2.
- Key result
- Compartment:
- natural water: freshwater
- DT50:
- 2 501 d
- Type:
- (pseudo-)first order (= half-life)
- Temp.:
- 20 °C
- Remarks on result:
- other: 10 µg/L
- Key result
- Compartment:
- natural water: freshwater
- DT50:
- 9 621 d
- Type:
- (pseudo-)first order (= half-life)
- Temp.:
- 20 °C
- Remarks on result:
- other: 100 µg/L test material
- Transformation products:
- no
- Remarks:
- For both concentrations no metabolite was formed during the incubation period in the water system.
- Conclusions:
- The DT50 values for [14C]test material were determined to be 2501 days (10 μg/L) and 9621 days (100 μg/L) in the water phases.
- Executive summary:
The rate of degradation of the test material, and the number and quantity of formed metabolites, were investigated in a study which was conducted in accordance with the standardised guideline OECD 309, under GLP conditions.
The study was performed under aerobic conditions in the dark using natural aerobic surface water taken from a large water body. To be able to determine the degradation rate and to follow the transformation products two different application rates (10 and 100 μg/L) of radio labelled test material were applied.
The DT50 values for [14C]test material were determined to be 2501 days (10 μg/L) and 9621 days (100 μg/L) in the water phases.
- Endpoint:
- biodegradation in water: sediment simulation testing
- Type of information:
- read-across from supporting substance (structural analogue or surrogate)
- Adequacy of study:
- key study
- Justification for type of information:
- Please see the read-across justification report in Section 13 of the dossier.
- Reason / purpose for cross-reference:
- read-across source
- Compartment:
- natural water / sediment: freshwater
- % Recovery:
- 96.9
- Remarks on result:
- other: Calwich Abbey system
- Compartment:
- natural water / sediment: freshwater
- % Recovery:
- 99.7
- Remarks on result:
- other: Swiss Lake system
- Key result
- Compartment:
- natural water / sediment: freshwater
- DT50:
- 83.2 d
- Type:
- other: Hockey stick model
- Temp.:
- 20 °C
- Remarks on result:
- other: Calwich Abbey system
- Key result
- Compartment:
- natural water / sediment: freshwater
- DT50:
- 244 d
- Type:
- other: Simpe first order
- Temp.:
- 20 °C
- Remarks on result:
- other: Swiss Lake system
- Transformation products:
- not specified
- Remarks:
- No intermediate metabolites were detected at, or above, an amount representing 5 % of the applied test material
- Conclusions:
- Under the conditions of this study, [14C]-test material in natural water/sediment systems was shown to degrade ultimately to carbon dioxide and unextractable sediment bound residues.
- Executive summary:
The route and rate of degradation of the test material in two water/sediment systems was investigated in accordance with the standardised guideline OECD 308 under GLP conditions.
The route and rate of degradation of [14C]-test material has been investigated under aerobic conditions at 20 ± 2 °C in two contrasting water sediment systems in the dark. The water sediment systems were Calwich Abbey [Staffordshire, UK, silt loam sediment, pH 7.2, 5.0 % OC] and Swiss Lake [Derbyshire, UK, loamy sand sediment, pH 6.6, 0.7 % OC].
Under the conditions of this study, [14C]-test material in natural water/sediment systems was shown to degrade ultimately to carbon dioxide and unextractable sediment bound residues.
- Endpoint:
- biodegradation in water: sediment simulation testing
- Type of information:
- read-across from supporting substance (structural analogue or surrogate)
- Adequacy of study:
- supporting study
- Justification for type of information:
- Please see the read-across justification report in Section 13 of the dossier.
- Reason / purpose for cross-reference:
- read-across source
- Compartment:
- water
- DT50:
- 38 d
- Type:
- other: P1 DissT50 (SFO)
- Compartment:
- sediment
- DT50:
- 39 d
- Type:
- other: P1 DissT50 (FOMC)
- Compartment:
- entire system
- DT50:
- 46 d
- Type:
- other: P1 DegT50 (SFO)
- Conclusions:
- The data did not allow a firm relationship to be built-up between the partitioning of test material to the sediment and degradation in the water phase in a two-compartment analysis. This was primarily a function of limited back-transfer from the sediment back into the water column.
- Executive summary:
The report covers the re-analysis of data from a previous study of the behaviour of the test material in water-sediment systems, according to the latest guidelines, in order to provide the most appropriate data for triggers for further studies and for inputs into environmental models of pesticide fate, specifically for use in FOCUS STEPS 1-4 for surface water modelling (FOCUS 2001, 2012).
FOCUS (2006) guidance on kinetics was used to evaluate two water-sediment datasets, Manningtree and Ongar. Two ‘Levels’ of analysis were used: Level P1, looking at a single compartment model and Level P2, looking at a two-compartment model with transfer between the water and sediment and vice versa.
The kinetic analysis conducted shows some differences to that originally performed. The values for Manningtree for both water and the whole system are similar to those previously reported. However, the values for Ongar for both water and the whole system are higher than those originally reported. The cause of the differences is likely due to the preparation of the data for analysis (exclusion of outliers) and the use of an SFO kinetics model rather than the three-compartment decay model used in the original analysis. This results in conservative degradation kinetic values for use in environmental fate modelling.
The data did not allow a firm relationship to be built-up between the partitioning of test material to the sediment and degradation in the water phase in a two-compartment analysis. This was primarily a function of limited back-transfer from the sediment back into the water column. In practice, this means that whole system DegT50 must be used in FOCUS STEP 3 modelling for one compartment (water) and the default DT50 of 1000 days used for the other compartment (sediment). This allocation is driven by the KOC of the test material as recommended by FOCUS (2012) guidance.
- Endpoint:
- biodegradation in water: sediment simulation testing
- Type of information:
- read-across from supporting substance (structural analogue or surrogate)
- Adequacy of study:
- supporting study
- Justification for type of information:
- Please see the read-across justification report in Section 13 of the dossier.
- Reason / purpose for cross-reference:
- read-across source
- Compartment:
- natural sediment
- % Recovery:
- 110
- Remarks on result:
- other: Aquatic sediment 96/03
- Compartment:
- natural sediment
- % Recovery:
- 106.2
- Remarks on result:
- other: Aquatic sediment 96/04
- Compartment:
- natural water
- DT50:
- 49.23 d
- Type:
- not specified
- Temp.:
- 20 °C
- Remarks on result:
- other: System 96/03
- Compartment:
- entire system
- DT50:
- 60.66 d
- Type:
- not specified
- Temp.:
- 20 °C
- Remarks on result:
- other: System 96/03
- Compartment:
- natural water
- DT50:
- 24.25 d
- Type:
- not specified
- Temp.:
- 20 °C
- Remarks on result:
- other: System 96/04
- Compartment:
- entire system
- DT50:
- 22.97 d
- Type:
- not specified
- Temp.:
- 20 °C
- Remarks on result:
- other: System 96/04
- Transformation products:
- not measured
- Volatile metabolites:
- yes
- Conclusions:
- Under the conditions of the study the test material is readily degraded in the aerobic aquatic systems and is therefore unlikely to persist in the aquatic environment.
- Executive summary:
The rate of test material degradation in two aquatic sediment systems was assessed according to BBA Guidelines, Part IV, Section 5-1 and in compliance with GLP.
The degradation of [14C]-test material, applied at a rate equivalent to 1.123 kg ai ha^-1, has been studied in two different aquatic sediment systems over a period of 100 days at 20 °C. The two sediments differed in that the Manningtree sediment had higher organic carbon and nitrogen content, whilst the Ongar system was more alkaline with a much higher cation exchange capacity.
The incubation was performed in glass flasks (approximately 7.5 cm internal diameter), containing sediment to an approximate depth of 2.5 cm covered with associated water to an approximate depth of 5 cm above the sediment. The units were maintained in the dark at 20 °C ± 2 °C. The water/sediment systems were incubated for approximately 6 weeks to enable acclimatisation, prior to [14C]-test material application to the surface water.
Moistened carbon dioxide-free air was supplied under positive pressure through the water in each unit and the effluent air passed through an ethylene glycol and two 2M potassium hydroxide traps, to trap any volatile products and liberated carbon dioxide (14CO2) respectively.
A good recovery of radioactivity was obtained for both systems with an overall mean recovery of 110.0 % (range 114.0 - 103.7 %) for system 96/03 (Manningtree). And 105. 5% (range 112.6 - 87.4 %) for system 96/04 (Ongar).
There was a steady transfer of the radioactivity from the water to the sediment in both systems, commencing at 111.29 % in the water phase at zero time and declining to 15.43 % (Manningtree system) and 1.80 % (Ongar system) after 100 days.
Extractable residues rose from 0 % at the first time-point (no extraction was made) to a maximum of 13.48 % at 14 days (Manningtree system). The Ongar system reached a maximum extracted residue level of 6.64 % after 14 days. Unextactable residues in both systems fluctuated with time from 0.77 % (Manningtree system) and 2.80 % (Ongar system) at time zero to 27.98 % (Manningtree system) at 100 days and 39.67 % (Ongar system) at 61 days.
Volatiles characterised as carbon dioxide accounted for 55 % (range 79 - 31 %) (Manningtree system) and 58 % (range 42 - 73 %) (Ongar system) of the applied dose after 100 days. Volatile materials, trapped in ethylene glycol, accounted for a negligible fraction (< 0.1 %) of the applied dose after 100 days. The test material was found to be the main component present in both the water and sediment phases. In addition significant mineralisation to carbon dioxide occurred. Some minor metabolites were observed in both the sediment and water phases, none of which exceeded 10 % of applied radioactivity in the total system.
Manningtree sediment: Water phase
DT50 = 49.23 days
DT90 = 155.47 days
Manningtree sediment: Complete system
DT50 = 60.66 days
DT90 = 175.23 days
Ongar sediment: Water phase
DT50 = 24.25 days
DT90 = 33.27 days
Ongar sediment: Complete phase
DT50 = 22.97 days
DT90 = 26.42 days
Under the conditions of the study the test material is readily degraded in the aerobic aquatic systems and is therefore unlikely to persist in the aquatic environment.
- Endpoint:
- biodegradation in water: simulation testing on ultimate degradation in surface water
- Type of information:
- read-across from supporting substance (structural analogue or surrogate)
- Adequacy of study:
- supporting study
- Justification for type of information:
- Please see the read-across justification report in Section 13 of the dossier.
- Reason / purpose for cross-reference:
- read-across source
- Remarks on result:
- not measured/tested
- Transformation products:
- not measured
- Conclusions:
- Under the conditions of the study the concentrations of the test material were reduced during the 50 - 129 d of incubation in both experiments. The data fitted approximate first-order kinetics without a lag phase.
The data indicate the test material is relatively stable in the aquatic environment whereas it degrades relatively quickly in soil (half-life, 7 - 22 d). The data for the test material show an approximately constant concentration (55 ng/L; lag phase) up to 21 d, followed by a gradual decrease to ≈ 11 ng/L at 89 d, the largest decrease occurring between 21 and 63 d. - Executive summary:
The degradation of the test material in surface water was determined in surface water collected from five lakes and two rivers situated in the central and eastern region of Switzerland.
The water from Sempachersee was incubated in 1 L green glass bottles and incubated for up to 129 d at room temperature (20 - 23 °C). Periodically, one of the bottles was removed from storage, and the water was analysed.
The water from the river Aabach was incubated in one batch in a 2.5-L clear glass bottle for up to 50 d at room temperature while being stirred with a Teflon bar. Periodically, 0.25 L subsamples were removed and analysed. From the amounts of oxygen dissolved and the relatively small amounts of dissolved organic matter, it can be assumed that the incubation conditions in both experiments were aerobic.
There was some algal growth observed in the clear glass bottle.
Additionally, incubation experiments were carried out with fortified water, using water from Sempachersee and the river Aabach. One 2.5-L batch of each were fortified at 50 - 100 ng/L with the test material.
The waters were incubated at room temperature in amber bottles for up to 89 d. Periodically, 0.25 L subsamples were removed and analysed. The fortifications were made by adding 1.0 mL of a 250 ng/ mL solution of the compounds in distilled water, prepared from stock solutions of the compounds in methanol.
Extraction from the acidified (pH ≈ 2) water was effected with a reusable small column containing a macroporous polystyrene adsorbent, and the analytes were eluted and recovered. After methylation with diazomethane, the extracts were concentrated (≈0.5 mL in diethyl ether) and passed through a small silica column (0.7 g of silica gel 60, deactivated with 5 % water; 5 mm i.d. Pasteur pipet) topped with 10 mm of sodium sulfate. The analytes (as methyl esters) were eluted with 10 mL ofn-hexane-methylene chloride (1:1). After careful concentration and dilution to 100 - 200μL withn-hexane, 2 μL aliquots were used for analysis by high-resolution gas chromatography/mass spectrometry (HRGC/MS).
Under the conditions of the study the concentrations of the test material were reduced during the 50 - 129 d of incubation in both experiments. The data fitted approximate first-order kinetics without a lag phase.
The data indicate the test material is relatively stable in the aquatic environment whereas it degrades relatively quickly in soil (half-life, 7 - 22 d). The data for the test material show an approximately constant concentration (55 ng/L; lag phase) up to 21 d, followed by a gradual decrease to ≈ 11 ng/L at 89 d, the largest decrease occurring between 21 and 63 d.
- Endpoint:
- biodegradation in water and sediment: simulation testing, other
- Remarks:
- biodegradation in water and sediment
- Type of information:
- read-across from supporting substance (structural analogue or surrogate)
- Adequacy of study:
- supporting study
- Justification for type of information:
- Please see the read-across justification report in Section 13 of the dossier.
- Reason / purpose for cross-reference:
- read-across source
- Compartment:
- sediment
- % Recovery:
- 11
- Remarks on result:
- other: Krempe
- Compartment:
- sediment
- % Recovery:
- 8
- Remarks on result:
- other: Ohlau
- Compartment:
- entire system
- % Recovery:
- 0.4
- Remarks on result:
- other: Parent compound
- Compartment:
- other: CO2
- % Recovery:
- 80
- Compartment:
- natural water / sediment: freshwater
- DT50:
- 21 d
- Type:
- other: Degradation curve
- Compartment:
- natural water / sediment: freshwater
- DT50:
- 21 d
- Remarks on result:
- other: Degradation curve
- Other kinetic parameters:
- other: DT90: 28 days
- Transformation products:
- not specified
- Conclusions:
- Under the conditions of the study the degradation of the test material proceeded rather rapidly in both systems. The main degradation product was carbon dioxide, which after three months comprised more than 80 % of the radioactivity originally applied to the system. Within this time the amount of parent compound decreased to less than 0.4 %. At the end of the test period three months after application approximately 4 to 8 % of the radioactivity were found in humic acids in the systems, another 3 to 4 % in fulvic acids. Ca. 11 % were retained in one sediment, ca. 8 % only in the other.
A disappearance time (DT) 50 of 20 to 21 days was found, the DT 90 was 28 days. This low DT 90 value may be explained by the exponential growth of the microbial degraders after a lack phase in the beginning of the experiment. - Executive summary:
The degradation of the test material in sediment/water was assessed according to Pesticide Assessment Guidelines Subdivision N, § 162-4 and in compliance with GLP.
The degradation of the test substance [14 C]-test material, being labelled in the aromatic ring was investigated in two different water/sediment systems under aerobic conditions over a period of three months at 22 °C. 1.88 mg test material was applied per kg of the system.
For both systems a disappearance time (DT) 50 of 20 to 21 days was found, the DT 90 was 28 days.
- Endpoint:
- biodegradation in water and sediment: simulation testing, other
- Remarks:
- Biodegradation in groundwater and sediment.
- Type of information:
- experimental study
- Adequacy of study:
- supporting study
- Study period:
- not reported
- Reliability:
- 2 (reliable with restrictions)
- Rationale for reliability incl. deficiencies:
- study well documented, meets generally accepted scientific principles, acceptable for assessment
- Qualifier:
- no guideline followed
- Principles of method if other than guideline:
- The potential of a riparian fen to mineralise herbicides that could leach from an adjacent catchment area was investigated. Slurries were prepared from sediment and ground water collected from different parts of a wetland representing different redox conditions. The slurries were amended with O2, NO3^-, SO4^2-, and CO2, or CO2 alone as electron acceptors to simulate the in situ conditions and their ability to mineralise the herbicide test material. In addition, the abundance of bacteria able to utilise O2, NO3^-, SO4^2- + CO2 and CO2 as electron acceptors was investigated along with the O2–reducing and methanogenic potential of the sediment.
- GLP compliance:
- not specified
- Radiolabelling:
- yes
- Remarks:
- Radiolabelled and unlabelled test material.
- Oxygen conditions:
- aerobic/anaerobic
- Inoculum or test system:
- natural water / sediment
- Details on source and properties of surface water:
- - Details on collection: Ground water was collected from polyethylene piezometers nested at the stations using an immersion pump and silicone tubing.
- Storage conditions: Samples were stored at 4 °C until use.
- Analysis of ground water: Oxygen, pH, and conductivity were measured in the field in a flow cell mounted with electrodes. Phosphate was measured by a modified ascorbic acid method, nitrite was measured spectrophotometrically. Other anions were analysed by ion chromatography. Ammonium was measured as indophenol. Ferrous iron was measured spectrophotometrically. Methane was analysed by GC-FID. Alkalinity was determined by titration. The total organic carbon content of the sediment was determined by combustion. - Details on source and properties of sediment:
- The sediment type was sand at Stations 0 and 5, sandy peat at S9-I, and peat at S9-II. Sediment cores were collected using a stainless steel borer and the sediment immediately transferred to sterile air-tight containers, completely filling them.
- Duration of test (contact time):
- 473 d
- Initial conc.:
- ca. 0.25 µg/L
- Based on:
- test mat.
- Parameter followed for biodegradation estimation:
- O2 consumption
- Details on study design:
- RESPIRATION RATES
Aerobic respirationwas determined as oxygen consumption in 116-mL serum bottles sealed with butyl rubber stoppers. Approximately 10 g of wet sediment was added to five replicate bottles and incubated at 10 °C. A test tube containing 2 mL 1 M NaOH was inserted in each bottle to trap evolved CO2. The pressure in the bottles was measured daily for 1 wk using a pressure transducer with a digital readout voltmeter. The pressure transducer had a range of 0 to 15 psi (0 – 103 kPa) with an accuracy of 0.1 psi ± 2 %. Five empty bottles served as controls to compensate for variation in atmospheric pressure. Standard curves were produced by withdrawal of known amounts of air from the bottles.
Potential methanogenesis was determined in similar bottles amended with sediment corresponding to 15 g dry weight and 30 mL ground water. Hydrogen (12.5 mL) and Na-acetate (3.4 mM) were added as electron donors. The flasks were incubated at 10 °C and evolution of methane followed on a gas chromatograph for 45 d.
MINERALISATION STUDIES
For studies of herbicide mineralisation, 100 mL of a stock solution containing the radiolabelled and unlabelled test material in methanol was added to sterile flasks. This corresponded to a total of 0.75 µg herbicide per flask or ca. 25 µg/L ground water. The flasks contained 1667 Bq of test material. The methanol was allowed to evaporate before addition of the sediment. Flasks (100 mL) with ground glass joints were used for the aerobic experiments, while 116-mL butyl rubber stoppered serum bottles with aluminum crimp seals were used for the anaerobic experiments.
The slurries were prepared in triplicate using wet sediment corresponding to 10 g dry weight and 30 mL ground water sampled from the same depth as the sediment. The sediment–ground water slurries from site S0 were set up aerobically while those from site S5 were set up in an atmosphere of N2 with the addition of 10 mM KNO3. The slurries from sites S9-II and S9-I were set up anaerobically in an atmosphere of 30 % CO2 and 70 % N2. The flasks simulating sulfate-reducing conditions were amended with 0.3 mL stock solution of Na2SO4 to supply 10 mM SO4^2- in the slurries (not taking into account the water added with the wet sediment). Slurries simulating methanogenic conditions were not amended with additional electron acceptors.
To trap the 14CO2 generated by mineralisation of the radiolabelled test material, a test tube containing 1 mL 1 M NaOH was mounted vertically in the culture flasks held in place by a metal needle inserted into the butyl rubber stopper. The NaOH was changed at each sampling occasion. The NaOH solution in the aerobic flasks was sampled using a pipette in a flow chamber while that in the anaerobic flasks was sampled anaerobically through the stopper using a N2–flushed disposable syringe mounted with a valve and a 0.9- x 120-mm stainless steel needle. 10 mL OptiPhase HiSafe scintillation fluid was added to each sample and the radioactivity determined using a Wallac 1409 liquid scintillation counter. Calculations on cumulative mineralisation were corrected for quenching and background decay. The data were reported as the average 14CO2 evolution for the triplicate slurries.
Radiolabelled 14CH4 in the headspace at the end of the experiment was combusted and measured as 14CO2. A 5-mL sample of headspace was passed through the flame ionization detector of a gas chromatograph and trapped in ethanolamine and 2-methoxyethanol (1:7 v/v).
To check for 14CO2 in the headspace of the slurries, the samples were also injected while the flame ionisation detector was switched off. - % Degr.:
- 36
- Parameter:
- O2 consumption
- Sampling time:
- 473 d
- Remarks on result:
- other: S0
- % Degr.:
- 8
- Parameter:
- O2 consumption
- Sampling time:
- 473 d
- Remarks on result:
- other: S5
- % Degr.:
- 10
- Parameter:
- O2 consumption
- Sampling time:
- 473 d
- Remarks on result:
- other: S9-I
- % Degr.:
- 13
- Parameter:
- O2 consumption
- Sampling time:
- 473 d
- Remarks on result:
- other: S9-II
- Transformation products:
- not measured
- Details on results:
- GEOCHEMISTRY AND MICROBIOLOGY
Analysis of the water chemistry at the sample sites verified that the ground water became increasingly reduced as it flowed toward the recipient stream and that sediment and ground water samples in fact represented different redox zones. Oxygen at aerobic levels was only detected at site S0, located on the hillslope. Sites S0 and S5 closest to the ground water recharge were rich in nitrate (> 1 mM), whereas sites S9-I and S9-II further along the transect were anaerobic and depleted of nitrate. The sulfate concentration was 927 µM at S9-I but only 94 M at S9-II, where methane was observed at a concentration of 24 mM. The presence of ammonium and ferrous iron at S9-I and S9-II further indicated the reduced state of these sites.
BACTERIAL ABUNDANCE AND RESPIRATION RATES
Aerobic bacterial abundance was one order of magnitude greater in the aerobic sediment (S0: 5.8 x 10^6 CFU) than in the denitrifying sediment (S5: 6.4 x 10^5 CFU). The abundance of denitrifying bacteria was lower than that of aerobic bacteria under both aerobic (S0) and denitrifying (S5) conditions. The number of bacteria using the prevalent electron acceptors was lower in the sulfate-reducing (S9-I) and methanogenic (S9-II) sediment than in the aerobic (S0) and denitrifying (S5) sediment. Both sulfate-reducing and methanogenic bacteria were more abundant in the methanogenic sediment (S9-II) than in the sulfate-reducing sediment (S9-I). However, the potential rates of methanogenesis did not differ significantly between these two sediments.
MINERALISATION
The test material was readily mineralised and was mineralised under both aerobic and anaerobic conditions. Under aerobic conditions (S0 slurries) mineralisation started immediately, and 36 % of the test material had mineralised by the end of the experiment, 473 d later. Under anaerobic conditions (S5 slurries), in contrast, only 8 % of the test material mineralised during the course of the experiment. Similarly low rates of mineralisation were obtained under both sulfate-reducing (S9-I: 10 %) and methanogenic conditions (S9-II; 13 %). Moreover, mineralisation of the test material in both S9-I and S9-II slurries was independent of the presence of added sulfate since the omission of sulfate from S9-I slurries and the addition of sulfate to S9-II slurries did not significantly affect the mineralisation of the test material. Also, there was no significant difference between the amounts of accumulated 14CO2 from test material in anaerobic slurries amended with nitrate and anaerobic slurries with sulfate or without additional electron acceptor. The presence of anaerobic conditions in the S9-I and S9-II slurries was confirmed in two ways.
(i) To ensure that oxygen had not entered the slurries during sampling, methane was measured in the culture flasks that had been sampled during the experiment and compared with unsampled controls. There was no significant difference in methane concentration in the sampled and unsampled slurries except for the S9-II slurries containing atrazine. Hence, methanogenesis was not inhibited due to oxygen entering the slurries during sampling.
(ii) Radiolabelled methane in the headspace of slurries was transformed to 14CO2 and measured. Approximately 0.1 to 1.5 % of the 14C initially added as [ring-U-14C]test material was recovered as 14CH4 after 460 d, corresponding dealky to 1.0 to 12.3 % of the accumulated 14CO2. - Validity criteria fulfilled:
- not applicable
- Conclusions:
- The test material was readily mineralised and was mineralised under both aerobic and anaerobic conditions. Under aerobic conditions (S0 slurries) mineralisation started immediately, and 36 % of the test material had mineralised by the end of the experiment, 473 d later. Under anaerobic conditions (S5 slurries), in contrast, only 8 % of the test material mineralised during the course of the experiment. Similarly low rates of mineralisation were obtained under both sulfate-reducing (S9-I: 10 %) and methanogenic conditions (S9-II; 13 %).
- Executive summary:
The potential of a riparian fen to mineralise herbicides that could leach from an adjacent catchment area was investigated. Slurries were prepared from sediment and ground water collected from different parts of a wetland representing different redox conditions. The slurries were amended with O2, NO3^-, SO4^2-, and CO2, or CO2 alone as electron acceptors to simulate the in situ conditions and their ability to mineralise the herbicide test material. In addition, the abundance of bacteria able to utilise O2, NO3^-, SO4^2- + CO2 and CO2 as electron acceptors was investigated along with the O2–reducing and methanogenic potential of the sediment.
Study findings revealed that aerobic bacterial abundance was one order of magnitude greater in the aerobic sediment (S0: 5.8 x 10^6 CFU) than in the denitrifying sediment (S5: 6.4 x 10^5 CFU). The abundance of denitrifying bacteria was lower than that of aerobic bacteria under both aerobic (S0) and denitrifying (S5) conditions. The number of bacteria using the prevalent electron acceptors was lower in the sulfate-reducing (S9-I) and methanogenic (S9-II) sediment than in the aerobic (S0) and denitrifying (S5) sediment. Both sulfate-reducing and methanogenic bacteria were more abundant in the methanogenic sediment (S9-II) than in the sulfate-reducing sediment (S9-I). However, the potential rates of methanogenesis did not differ significantly between these two sediments.
Under the conditions of the study, the test material was readily mineralised and was mineralised under both aerobic and anaerobic conditions. Under aerobic conditions (S0 slurries) mineralisation started immediately, and 36 % of the test material had mineralised by the end of the experiment, 473 d later. Under anaerobic conditions (S5 slurries), in contrast, only 8 % of the test material mineralised during the course of the experiment. Similarly low rates of mineralisation were obtained under both sulfate-reducing (S9-I: 10 %) and methanogenic conditions (S9-II; 13 %). Moreover, mineralisation of the test material in both S9-I and S9-II slurries was independent of the presence of added sulfate since the omission of sulfate from S9-I slurries and the addition of sulfate to S9-II slurries did not significantly affect the mineralisation of the test material. Also, there was no significant difference between the amounts of accumulated 14CO2 from test material in anaerobic slurries amended with nitrate and anaerobic slurries with sulfate or without additional electron acceptor.
- Endpoint:
- biodegradation in water and sediment: simulation testing, other
- Remarks:
- biodegradation in water and sediment
- Type of information:
- experimental study
- Adequacy of study:
- supporting study
- Study period:
- June 1990 to August 1991
- Reliability:
- 2 (reliable with restrictions)
- Rationale for reliability incl. deficiencies:
- comparable to guideline study with acceptable restrictions
- Reason / purpose for cross-reference:
- other: read-across target
- Qualifier:
- according to guideline
- Guideline:
- EPA Subdivision N Pesticide Guideline 162-4 (Aerobic Aquatic Metabolism)
- Deviations:
- no
- GLP compliance:
- yes
- Radiolabelling:
- yes
- Oxygen conditions:
- aerobic
- Inoculum or test system:
- natural water / sediment: freshwater
- Details on source and properties of surface water:
- - Details on collection: Two different water-sediment systems (soil and water each) were collected from the area north-west and north of Hamburg on June 27, 1990.
stream "Grosser Graben", near Krempe/Elmshorn in a marsh area surrounded by arable land and pastures; in this report called "Krempe".
stream "Ohlau", near Lentforden surrounded by pastures; in this report called "Ohlau".
- Storage length: Water parameters were determined within 23 h after collecting the samples, after the sediment had settled.
- pH at time of collection: Krempe: 7.6; Ohlau 7.7
- Oxygen concentration (mg/l) initial/final: Krempe: 7.5 mg/L initial; Ohlau 5.3mg/L initial. - Details on source and properties of sediment:
- - Details on collection: Two different water-sediment systems (soil and water each) were collected from the area north-west and north of Hamburg on June 27, 1990.
stream "Grosser Graben", near Krempe/Elmshorn in a marsh area surrounded by arable land and pastures; in this report called "Krempe".
stream "Ohlau", near Lentforden surrounded by pastures; in this report called "Ohlau".
- Textural classification: Krempe: loamy-sandy; Ohlau: Sandy
- pH at time of collection: Krempe: 6.2; Ohlau 6.9; determined in 0.01 m CaCl 2 solution.
- Organic carbon (%): Krempe: 1.85; Ohlau: 0.25
- CEC (meq/100 g): Krempe: 8; Ohlau: 18
- Particle size Krempe:
< 0.002 mm: 1.2
0.002 - 0.020 mm: 1.0
0.006 - 0.060 mm: 1.0
0.020 - 0.060 mm: 6.1
0.060 - 0.200 mm: 41.0
0.200 - 0.600 mm: 46.9
0.600 - 2.0 mm: 2.8
- Particle size Ohlau:
< 0.002 mm: 3.0
0.002 - 0.020 mm: 1.0
0.006 - 0.060 mm: 2.8
0.020 - 0.060 mm: 6.9
0.060 - 0.200 mm: 35.4
0.200 - 0.600 mm: 49.1
0.600 - 2.0 mm: 1.8 - Duration of test (contact time):
- 3 mo
- Initial conc.:
- 1.88 other: mg/kg
- Based on:
- test mat.
- Parameter followed for biodegradation estimation:
- radiochem. meas.
- Details on study design:
- Radioactivity measurements
Determination of radioactivity in liquid samples
Aliquots of the liquid samples (solutions) in amounts up to 1 mL were weighed into glass scintillation vials and scintillation cocktail (Hionic Fluor (R) of Packard Instrument GmbH, Frankfurt, FRG) was added. In general, threefold samples were prepared from each solution.
The radioactivity was then determined in a liquid scintillation spectrometer, model Tri-Carb 4530 of Packard Instrument GmbH according to a standard operation procedure.
From the dpm values obtained the specific and total radioactivities were calculated under consideration of the aliquot used for the measurements
Calculation of specific radioactivities and equivalents of the test material
The basis for conversion of dpm’s into kBq or Bq was: 1 kBq ~ 60 000 dpm or 1 Bq = 60 dpm respectively.
Specific radioactivities in liquids and solids were calculated from the following expression:
Specific radioactivity (Bq/g) = dpm / (60 x m)
where m is the weight (in g) of the aliquot of liquid or solid used for the radioactivity determination.
From the individual values the mean value and the standard deviation were calculated.
The total radioactivity (At) contained in solution or solid was calculated from the specific radioactivities by multiplication with the respective weights. This was related to the radioactivity originally applied (Aa) to the test system resulting in the percentage of radioactivity contained in the respective solution or solid.
Percentage = (At [kBq] / Aa [kBq]) · 100
Combustion of dried sediment samples
Aliquots of the sediment samples were combusted after extraction and drying in a Tri-Carb sample oxidiser model 306 of Packard Instrument GmbH, Frankfurt, FRG according to a standard operation procedure. In general, five parallel samples were combusted per sample. The radioactivity in the resulting samples was determined by liquid scintillation counting.
Microbiological investigations
The total germ count was determined according to Deutsche Einheitsverfahren zur Wasser-, Abwasser- und Schlammuntersuchung, mikrobiologische Verfahren (Gruppe K) DIN 38411, Teil 5) method K 5, omitting the membrane filtration.
Degradation of the test material
Preparation of the solution of [14C]-test material to be applied to the test system
Unlabelled test material and [14C]-test material were dissolved in methanol, resulting in a concentration of 2.2 mg (14 C-labelled)-test material per mL.
Test procedure
For investigation of the degradation of the test material the general procedure was followed as outlined in our drafted standard operation procedure (SOP).
Test device
The incubation flask (volume ca. 1.3 L) is equipped with a device for slow stirring of the water only, a trap for volatile components and a tube for introducing air. The trap, containing quarz wool (being impregnated with paraffin oil) and soda lime, is permeable to air. Slow stirring of the water is accomplished by placing the flask on a shaking cupboard with revolving movement. Thus, aerobic conditions are achieved in the water. Dissolved oxygen, temperature and pH are measured at each sampling date.
Preparation of the flasks
A total of 12 flasks was prepared per system, each containing 55 g (dry weight) soil and 550 mL water. They were placed on the shaking cupboard and allowed to equilibrate for 2 weeks before the 14C-labelled test material was applied.
Application of the test material
0.5 mL each of the methanol solution of [14C]-test material were applied to 10 of the 12 flasks prepared. For determination of the amount of test material applied to the flasks, three replicate applications were done in the same way into methanol. The radioactivity in these solutions was determined via LSC. Thus 1.14 mg or 905.6 kBq of the material were present in the flask, corresponding to a concentration of 1.88 mg per kg system. No test material was applied to both flasks allocated to monitoring of the biological system.
Sampling and extraction procedure
At each sampling 50 mL water were withdrawn from the system for determination of O2 content, pH and temperature. Then volatile compounds were driven from the headspace of the flask into the trap by a stream of air (10 min, 10 mL/sec); subsequently the aqueous layer in the flask was set to approximately pH 10 with aqueous 1 N KOH and the contents of the flasks was transferred into centrifuge vials; the portion of water previously withdrawn for determination of the water parameters was used for rinsing and thus added to the system again.
After separation of the layers by centrifugation an aliquot of the aqueous phase was acidified (pH 1) with aqueous 1N HCl and extracted with dichloromethane (5 times, 30 mL each). After determination of the radioactivity in the combined solution it was investigated by means of radio-TLC.
Another aliquot of the aqueous layer was acidified in the same way and in a closed flask, heated to 70 °C
for 30 min during which time a slight stream of nitrogen was passed through the solution and trapped in an absorption solution for CO2. The radioactivity in this solution was determined.
The sediment was extracted with methanol/water (1:1) followed by methanol. After determination of the radioacitivity the solutions were combined and investigated via radio-TLC.
After drying, the radioactivity retained in the sediment was determined by means of combustion/LSC.
Carbonate in extracted sediment samples
For determination of the amounts of 14C-labelled carbonate, an aliquot of the sediment was suspended in aqueous HCl (pH 1) in a closed system and during stirring and heating (70 °C) a slight stream of nitrogen was driven over this solution and passed through an absorption liquid for carbon dioxide. The radioactivity in this solution was determined.
Investigation of extracted sediment samples
An aliquot (ca. 5 g) of the dry sediment was extracted three times with 20 mL 0.1 N NaOH each with ultrasonication and washed with water. After determination of the radioactivity in the alkaline solution this was acidified with 1 N HCl to pH 1.
The precipitate formed was separated by centrifugation and washed with 0.1 N HCl. The radioactivity was determined in the acidic solution, in the precipitate (after dissolution in 0.1 N NaOH) and in the dried sediment. - Reference substance:
- other: 2-Methyl-4-chlorophenol
- Compartment:
- sediment
- % Recovery:
- 11
- Remarks on result:
- other: Krempe
- Compartment:
- sediment
- % Recovery:
- 8
- Remarks on result:
- other: Ohlau
- Compartment:
- entire system
- % Recovery:
- 0.4
- Remarks on result:
- other: Parent compound
- Compartment:
- other: CO2
- % Recovery:
- 80
- Compartment:
- natural water / sediment: freshwater
- DT50:
- 21 d
- Type:
- other: Degradation curve
- Other kinetic parameters:
- other: DT90: 28 days
- Transformation products:
- not specified
- Evaporation of parent compound:
- yes
- Volatile metabolites:
- yes
- Residues:
- not specified
- Details on results:
- The degradation of the test material in two different water/ sediment systems was investigated under aerobic conditions using radioanalytical methods. The sediments employed, named "Krempe" and “Ohlau", were a loamy-sandy and a sandy in character, respectively.
1.14 mg [ring-u-14C)-test material dissolved in 0.5 mL methanol were applied to each flask containing 55 g (dry weight) sediment and 550 mL water, being equivalent to an application rate of 1.88 mg per kg system. According to TLC-investigation in two systems the radiochemical purity of the test material applied was 87 to 88 %. This figure was got by a real worst-case estimation, when every count was considered. By this the impurities were split into 15 separate peaks, the most important of which accounted for no more than 2 - 3 % each.
The study was conducted at 22 ± 2 °c in the dark over 92 days with 7 samplings at 0, 3, 7, 14, 30, 60 and 91/92 days after application of the test material (the last sampling for the system Krempe was done 91 dafter application, whereas this was done for the system Ohlau after 92 d).
Water parameters
Temperature, pH and O2-concentration were determined in the flask at each sampling date. For the system Ohlau, a slight decrease in pH was observed from day 30 to day 92. The o2-concentration started between 6 and 7 mg/L, after a slight increase at day 3 it dropped to 3.8 mg/L in the system Krempe at day 7 and subsequently recovered to more than 8 mg/L. This decrease in oxygen concentration was paralleled by some turbidity in the systems which was observed starting after ca. day 7 and reaching the maximum at ca. day 10; subsequently it slowly disappeared. This turbidity was not observed in the sample for the control of the biological system, to which no test material had been applied.
However, the data showed that there were always aerobic conditions in the water phases.
The total germ number in the water was determined within 20 h after collecting the system from the respective source and during the experiment on a water/sediment sample which was contained in a degradation flask but which contained no test material. The total germ counts after collecting the system were approximately 7 x 10^3 in the Krempe water and approximately 3 x 10^5 in the Ohlau water. The counts decreased to ca. 20 00 (Krempe) or ca. 3 600 (Ohlau) respectively within ca. two weeks until application and further decreased slightly until the end of the experiment.
During development of the turbidity in the water the total germ count was determined in a Krempe sample to which 14C-labelled test material had been applied. On day 10 after application the germ count was 5.9 x 10^6, on day 18 it was 5.8 x 10^4 . This finding indicates that the microorganisms involved in the breakdown of the herbicide used the solvent (methanol) or the active ingredient as a carbon source.
Radioactivity in the different parts of the system
The data show that in both systems Krempe and Ohlau between day 3 and day 14 after application of the substance the distribution of radioactivity in the different parts was found to be rather constant: 81 to 85 % of the radioactivity applied were found in the water, 14 to 18 % in the sediment-and at most 4 % in the volatiles. Between day 14 and day 91/92 (Krempe/Ohlau) the percentage in the volatiles, being carbon dioxide increased rapidly to 83 % (Krempe) or to 80 % (Ohlau) whereas the amount in the water decreased to 3 % or less. The amount in the sediment increased to 19 % (Krempe) or to 29 % (Ohlau). But whereas in the beginning most of the radioactivity was extractable from the sediment into methanol/water, at the end most of it was retained.
Identity of the radioactivity
As far as significant amounts of radioactivity were found in the water (until day 14 after application) most of this was extractable into dichloromethane. For TLC investigation of these latter extract solutions different TLC systems were examined. Radio-TLC investigation of the dichloromethane extracts mentioned revealed that almost all of the radioactive residue consisted of parent [14C]-test material. Some more parent compound could be extracted from the sediment and identified via radio-TLC as well. Thus, the total percentage of parent compound found in both systems until day 14 was more than 83 %, related to the amount applied. After day 14 the amount of parent compound decreased rapidly to 0.4 % or less.
No significant amounts of 2-methyl-4-chloro-[14C]-phenol as potential metabolite could be identified.
After day 14 up to 83 % of the radioactivity applied were found directly in volatile compounds which could be trapped with soda lime and thus were assigned as carbon dioxide. Minor amounts of carbon dioxide or carbonate were additionally found in the water or in the sediment respectively.
From the data it is concluded that at day 30, when significant amounts of carbon dioxide had been formed, slight amounts of volatile radioactivity - probably carbon dioxide - were lost during manipulation of water until determination of the CO2. For this reason, from day 60 the aqueous layer was set slightly alkaline after sweeping the volatile compounds into the trap and before opening the degradation flask. This resulted in an improved recovery of radioactivity at least for the system Krempe.
Radioactivity retained in the sediment
After extraction of the sediment with methanol in the samples of day 30 to day 91/92 radioactivity was retained therein in amounts of 11 to 26 %. For characterising this radioactivity the sediment samples were extracted with aqueous sodium hydroxide. This extract was subsequently acidified. It could be demonstrated that roughly half of the radioactivity from the sediment was extractable into aqueous sodium hydroxide being equivalent to 7 to 12 % (Krempe) or to 7 to 15 % (Ohlau) related to the amount applied. For the samples "day 91/92" approximately 4 or 8 % respectively of the radioactivity, to be considered as humic acids, were precipitated by hydrochloric acid (pH 1) whereas 3 to 4 % (fulvic acids) were soluble in the acidic aqueous solution. - Validity criteria fulfilled:
- not specified
- Conclusions:
- Under the conditions of the study the degradation of the test material proceeded rather rapidly in both systems. The main degradation product was carbon dioxide, which after three months comprised more than 80 % of the radioactivity originally applied to the system. Within this time the amount of parent compound decreased to less than 0.4 %. At the end of the test period three months after application approximately 4 to 8 % of the radioactivity were found in humic acids in the systems, another 3 to 4 % in fulvic acids. Ca. 11 % were retained in one sediment, ca. 8 % only in the other.
A disappearance time (DT) 50 of 20 to 21 days was found, the DT 90 was 28 days. This low DT 90 value may be explained by the exponential growth of the microbial degraders after a lack phase in the beginning of the experiment. - Executive summary:
The degradation of the test material in sediment/water was assessed according to Pesticide Assessment Guidelines Subdivision N, § 162-4 and in compliance with GLP.
The degradation of the test substance [14 C]-test material, being labelled in the aromatic ring was investigated in two different water/sediment systems under aerobic conditions over a period of three months at 22 °C. 1.88 mg test material was applied per kg of the system.
After an incubation time of approximately two weeks, the degradation of the test material proceeded rather rapidly in both systems. The main degradation product was carbon dioxide, which after three months comprised more than 80 % of the radioactivity originally applied to the system. Within this time the amount of parent compound decreased to less than 0.4 %.
At the end of the test period three months after application approximately 4 to 8 % of the radioactivity were found in humic acids in the systems, another 3 to 4 % in fulvic acids. Ca. 11 % were retained in one sediment, ca. 8 % only in the other.
For both systems a disappearance time (DT) 50 of 20 to 21 days was found, the DT 90 was 28 days. This low DT 90 value may be explained by the exponential growth of the microbial degraders after a lack phase in the beginning of the experiment.
- Endpoint:
- biodegradation in water: sediment simulation testing
- Type of information:
- experimental study
- Adequacy of study:
- supporting study
- Reliability:
- 2 (reliable with restrictions)
- Rationale for reliability incl. deficiencies:
- study well documented, meets generally accepted scientific principles, acceptable for assessment
- Qualifier:
- no guideline followed
- Principles of method if other than guideline:
- The natural attenuation of the test material was studied by determining changes in enantiomeric fraction in different redox environments down gradient from a landfill in the Lincolnshire limestone. In order to confirm the processes occurring in the field, microcosm experiments were undertaken using limestone acclimatised in different redox zones.
- GLP compliance:
- not specified
- Specific details on test material used for the study:
- Racemic mixture, deposited in a landfill in the Lincolnshire limestone aquifer.
- Radiolabelling:
- no
- Oxygen conditions:
- aerobic/anaerobic
- Inoculum or test system:
- other: natural groundwater / sediment
- Details on source and properties of surface water:
- Groundwaters for use in the laboratory microcosms were collected from six boreholes. Groundwater samples were collected using a Grundfos SP1 submersible pump, in 3 L polyethylene terephthalate (PET) bottles with no headspace. The PET bottles have an integral barrier layer polymer to preclude gas permeation.
- Details on source and properties of sediment:
- Microbially colonised sediments for use in the microcosms were prepared by suspending polypropylene mesh cylinders (Plastock Polymers, Birkenhead, UK) containing crushed Lincolnshire limestone (1 mm dia.) down the boreholes for three months. The limestone was obtained from a quarry in the vicinity of the landfill in the Lincolnshire limestone and correlated with the lithological horizon of the limestone in the aquifer.
- Duration of test (contact time):
- 224 d
- Parameter followed for biodegradation estimation:
- test mat. analysis
- Details on study design:
- MICROCOSM PREPARATION
Twenty four microcosms were constructed using standard, 250 mL amber-glass, reagent bottles. Twelve of these were fitted with screw-cap Mininert® valves to provide a set of anaerobic microcosms (i.e. six sterile and six non-sterile). The remaining 12 were reserved for a set of aerobic microcosms, and these were fitted with ordinary screw-caps containing PTFE-faced liners.
Anaerobic microcosms were assembled in a glovebox under an atmosphere of nitrogen. Moist sediment (ca. 50 g dry weight) was added to each bottle followed by 200 mL of the respective groundwater. Microcosms that were to be sterile controls also contained 0.4 g of sodium azide (i.e. 0.2 % w/v). The anaerobic microcosms were placed in stainless steel vessels, through which nitrogen could be flushed, and agitated gently on shakers installed in an incubator maintained at 10 ± 1 °C. Periodically the microcosms were taken from their nitrogen-filled containers and sampled by syringe. Septa on the Mininert® valves were intentionally cored so that nitrogen flowing across the face of the valve would be preferentially drawn into the microcosms at this point by the partial vacuum created on withdrawal of a sample.
Similar aerobic microcosms fitted with screw caps were prepared. Periodic sampling involved removing the screw-cap and taking a sample by syringe. After sampling, and before return to the incubator, headspace air in the aerobic microcosms was exchanged for fresh air to maintain aerobic conditions. - Transformation products:
- yes
- Remarks:
- (R-isomer degradation - anaerobic)
- No.:
- #1
- Details on results:
- Results from the individual microcosm experiments are discussed below.
1. Methanogenic/sulphate reducing microcosms (Borehole 28)
No degradation was observed in the methanogenic microcosms from Borehole 28. The EF remained at 0.50 ± 0.01 in both sterile and non-sterile microcosms throughout their 224 day operation, although there was some decline in test material concentration from 3 100 to 2 800 µg/L. Since this occurred in both sterile and non sterile microcosms, to the same extent, it was attributed to abiotic processes, possibly chemical reaction. The walls of the non-sterile microcosm developed a coating of black iron sulphide during the incubation and sulphate concentration declined from 105 to 12 mg/L in 119 days, indicating active sulphate reduction. This did not occur in the sterile microcosm.
It appears that in this microbially active environment, micro-organisms for mecoprop degradation are either not present, or are being inhibited, or are preferentially degrading other organic compounds.
After 119 days a nitrate amendment (543 mg/L) was made to assess the effect of this terminal electron acceptor on test material degradation. Although no degradation was observed the nitrate supplement did result in a steady increase in sulphate in the non-sterile microcosm from 12 mg/L at 119 days to 623 mg/L at 224 days. This was attributed to microbially mediated oxidation of both the black iron sulphide, observed on the surfaces of the sediment after acclimatisation, and that formed on the walls of the microcosm. The stoichiometry of this oxidation (3 moles of nitrate produce 2 moles of sulphate) corresponds to the observed decrease in nitrate concentration from 543 to 1 mg/L over the same period (i.e. the consumption of 542 mg/L nitrate led to the production of 611 mg/L sulphate). It is possible that no test material degraded because all the available nitrate was consumed in sulphide oxidation.
2. Nitrate-reducing microcosms (Borehole 32)
Initially, these microcosms contained 4 µg/L of R-isomer compared with 680 µg/L of S-isomer (EF = 0.01). Although the concentration of R-isomer was close to the detection limit there were indications that after six days the R-isomer had disappeared from the non-sterile microcosm but not from the sterile one. This implied either enantioselective anaerobic biodegradation of R-isomer or inversion of R-isomer to S-isomer. To confirm which, the microcosms were spiked with 500 µg/L racemic test material after 18 days. This resulted in an EF of 0.29 in the non-sterile microcosm and 0.31 in the sterile microcosm. Thereafter, in the non-sterile microcosm the EF fell to 0.28 after 3 days then to 0.13 after 13 days and was 0.00 after 17 days. No evidence of increasing concentration of S-isomer signifying (R)- to (S)-inversion was observed. EF in the sterile microcosm, which received no further test material amendments, remained at 0.31 ± 0.02 for the ensuing 161 days of the experiment. A further spike of R-isomer to the non-sterile microcosm, producing a concentration of 10 471 µg/L, was made 46 days after the microcosm was first started. After this amendment S-isomer remained constant at 1 263 ± 18 µg/L throughout, but that R-isomer was transformed with the concomitant growth of the metabolite 4-CMP. Constancy of S-isomer concentration, during the profound decrease in R-isomer concentration, confirmed that biodegradation and not chiral inversion was the process involved.
The maximum concentration of 4-CMP achieved was 6 856 µg/L which would have required stoichiometric conversion of 10 322 µg/L of test material which corresponds well with the initial concentration of 10 471 µg/L for R-isomer. Only when R-isomer disappeared did biodegradation of 4-CMP commence. This suggested that 4-CMP was a transient metabolite formed by cleavage of the ether linkage between the aromatic and propionic acid moieties of R-isomer.
A plot of R-isomer concentration against time produced a graph that gave a coefficient of regression (R^2), of 0.9733 when a linear fit was employed, indicating that degradation could be described by zero-order kinetics with a degradation rate of 0.65 mg/L/day. Whilst zero-order kinetics provide a reasonable fit to the data they do not imply any understanding of the complex kinetics of the underlying microbial processes and the extraction of a zero-order anaerobic degradation rate is intended merely to give some approximate idea of relative disappearance rates when comparing anaerobic and aerobic degradation in the microcosms.
Nitrate reduction was identified as the terminal electron accepting process for the following reasons. This groundwater (Borehole 32) had the highest nitrate concentration of all microcosm groundwaters studied at 29.9 mg/L. Nitrate concentration declined progressively throughout the experiment to below detection limit (< 0.2 mg/L) some 73 days after the final large R-isomer spike, (i.e. 119 days from initial construction of the microcosm), by which time all R-isomer and all 4-CMP had degraded. This then preceded the 119 day nitrate addition, 500 µg/L, that had been made to all anaerobic microcosms. Stoichiometric considerations reveal that 27.7 mg/L of nitrate would be required to completely mineralise the 10 898 µg/L of R-isomer that had been present in the microcosm since its construction and subsequent spikings. Nitrite, which is a transient species generated during microbial nitrate reduction, was detected only in the non-sterile microcosm during the experiment. It was not detected in the sterile microcosm.
Iron reduction may be ruled out because there was very little iron(II) in Borehole 32 groundwater at the start of the experiment (0.05 mg/L) and this concentration remained constant throughout. Had iron reduction occurred steadily increasing concentrations of aqueous iron(II) would have been expected due to reductive dissolution of insoluble iron(III) minerals. Likewise, there was no evidence of sulphate reduction since sulphate remained steady at 150 mg/L in both sterile and non-sterile microcosms.
3. Induced nitrate-reducing microcosms (Boreholes 4, 25 and 9)
Similar behaviour to that in Borehole 32 microcosms was found in some of the other anaerobic non-sterile microcosms (Boreholes 4, 25 and 9) after the 119 day nitrate addition. Thus, for Borehole 4 microcosm, degradation of R-isomer commenced promptly following addition. Microcosms for Boreholes 25 and 9 behaved analogously to the Borehole 4 microcosm, but with lag times of around 20 and 35 days, respectively, following nitrate amendment before degradation commenced and with maximum 4-CMP formation at 200 and 220 days from initial construction, respectively.
In common with Borehole 32, groundwaters from Boreholes 4, 25 and 9 had EF < 0.5, but, they also had lower nitrate concentrations; 12.0, 3.85 and < 0.6 mg/L, respectively. In each non-sterile microcosm assembled using these groundwaters nitrate content had been completely consumed after 53 days with no observable impact upon test material concentrations. It is interesting to note that lag periods associated with R-isomer biodegradation, following nitrate amendment, correlate with proximity of the boreholes to Borehole 32. Thus, Borehole 4, only 90 m down-flow from Borehole 32 displays, in common, no lag preceding test material biodegradation. For Borehole 25 which is about 500 m up-flow from Borehole 32 the lag period is about 20 days and for Borehole 9 situated about a kilometre down-flow the lag is approximately 35 days. Differences in lag times may indicate the relative degree of acclimatisation of micro-organisms responsible for enantioselective anaerobic R-isomer biodegradation at the various locations, i.e. reflect the length of time since they were actively engaged in this process.
4. Sulphate reducing microcosms (Borehole 35)
No perceptible changes in enantiomeric fraction throughout the 224 day duration of investigation were observed in the microcosm pair constructed with groundwater from the borehole situated furthest from the landfill (Borehole 35), 4.5 km down-gradient, in the confined sulphate-reducing region of the aquifer. Because this groundwater contained about 0.7 µg/L total test material and only produced measurable concentrations of test material after pre-concentration of a large volume by solid-phase extraction, it was amended with racemic mecoprop to produce microcosm concentrations of each enantiomer of 129 µg/L (non-sterile) and 138 µg/L (sterile), well above the HPLC limit of detection. For both sterile and non-sterile microcosms enantiomeric fraction remained at 0.50 ± 0.01 throughout, regardless of the 119 day nitrate amendment. NPOC was low at < 1.00 mg/L, less than at Borehole 9, situated 1.5 km from the landfill, where NPOC was 3.29 mg/L. Both these boreholes occur in the confined region of the aquifer, and have the lowest NPOC values of the six groundwaters examined, yet both show differing anaerobic test material-degrading behaviour. Thus, Borehole 9, following a lag period, yielded rapid anaerobic biodegradation of test material when sufficient nitrate was present, whereas nitrate would not induce Borehole 35 to react in this manner. However, Borehole 9 is much closer to the unconfined zone of the aquifer and its groundwater does not possess the sulphate-reducing characteristics of Borehole 35 groundwater which result from prolonged confinement. Lack of test material-degrading activity in Borehole 35, in contrast to Borehole 9, may be due to an oligotrophic environment in which no acclimatised test material-degraders are supported because of low test material and NPOC concentrations. Alternatively, it could be that the sulphate-reducing conditions found to prevail at Borehole 35 inhibit anaerobic test material degradation.
5. Aerobic microcosms (Boreholes 32, 4 and 9)
Microcosms, that had exhibited enantioselective biodegradation of only R-isomer when kept under anaerobic conditions, were able aerobically to biodegrade test material rapidly and enantioselectively but in a very different fashion. Non-sterile aerobic microcosms from Boreholes 32, 4 and 9 exhibited fast degradation of both test material enantiomers with no perceptible lag phase. To quantify biodegradation rates these three nonsterile microcosms were spiked with racemic mecoprop to give initial test material concentrations of about 15 000 µg/L. Zero-order rate constants and associated linear regression coefficients reveal faster biodegradation of S-isomer, with ultimate biodegradation of both enantiomers.
Rates obtained for aerobic degradation are significantly greater than those for anaerobic degradation, but it should be borne in mind that a constant plentiful supply of atmospheric oxygen was provided to all aerobic microcosms. Lack of a lag-phase suggests that the microorganisms responsible for aerobic transformation were acclimatised and had therefore at some recent stage been performing this function. It seems probable that in the vicinity of Boreholes 32, 4 and 9, where water level fluctuations show large seasonal variations redox conditions can shift from anaerobic to aerobic and vice versa, but the microbial community is capable of degrading test material under either condition.
6. Aerobic microcosms (Boreholes 25, 28 and 35)
The remaining non-sterile microcosms constructed with groundwaters from Boreholes 25, 35 and 28 also displayed aerobic test material biodegradation but only after significant and differing lag times of 20, 60 and 100 days respectively. Aerobic behaviour of the microcosm pair prepared from Borehole 25 groundwater was typical of degradation encountered with these three groundwaters. The lag-phase suggests that microorganisms responsible for aerobic transformation were present but were not acclimatised. No 4-CMP was detected in any aerobic microcosm chromatograms, probably because its transformation rate was equal to or greater than that of test material under the conditions prevailing in our experiments. - Validity criteria fulfilled:
- not applicable
- Conclusions:
- The laboratory microcosms confirmed the processes contributing to variations in enantiomeric fraction of test material down gradient from a landfill in a limestone aquifer.
They revealed that the test material does not degrade in methanogenic/sulphate-reducing and iron-reducing conditions. In nitrate-reducing conditions R-isomer degrades but S-isomer does not. In aerobic conditions S-isomer degrades at a faster rate than R-isomer. There was no indication of enantiomeric inversion.
It was also possible to show that anaerobic transformation proceeded by initial cleavage of the ether linkage leading to formation of the transient metabolite 4-CMP. Only when all R-isomer had been converted to 4-CMP did the metabolite itself begin to biodegrade anaerobically. - Executive summary:
The natural attenuation of test material was studied by determining changes in enantiomeric fraction in different redox environments down gradient from a landfill in the Lincolnshire limestone. Such changes could be due to differential metabolism of the enantiomers, or enantiomeric inversion. In order to confirm the processes occurring in the field, microcosm experiments were undertaken using limestone acclimatised in different redox zones.
No biodegradation was observed in the methanogenic, sulphate-reducing or iron-reducing microcosms. In the nitrate-reducing microcosm S-isomer did not degrade but R-isomer degraded with zero order kinetics at 0.65 mg/L/day to produce a stoichiometric equivalent amount of 4-chloro-2-methylphenol. Only when R-isomer disappeared did biodegradation of 4-CMP commence. This suggested that 4-CMP was a transient metabolite formed by cleavage of the ether linkage between the aromatic and propionic acid moieties of R-isomer.
The addition of nitrate to dormant iron-reducing microcosms devoid of nitrate stimulated anaerobic degradation of R-isomer after a lag period of about 20 days and was associated with the production of 4-chloro-2-methylphenol. Nitrate addition to sulphate-reducing/methanogenic microcosms did not stimulate test material degradation. However, the added nitrate was completely utilised in oxidising sulphide to sulphate. There was no evidence for enantiomeric inversion.
The study reveals new evidence for fast enantioselective degradation of R-isomer under nitrate-reducing conditions.
Referenceopen allclose all
Bacterial Density and Activity at the Aerobic and Denitrifying Sites
Site |
Aerobic Bacteria (CFU/g dry soil*) |
O2 Consumption (µmol/g dry soil/day) |
Denitrifying Bacteria (cells/g dry soil) |
0 |
5.8 x 10^6 |
0.36 |
< 7.0 x 10^5 |
5 |
6.4 x 10^5 |
nd |
4.2 x 10^5 |
* CFU: Colony forming units.
nd: Not determined
Bacterial Density and Activity at the Sulfate-Reducing and Methanogenic Sites
Site |
Sulfate-Reducing Bacteria (cells/g dry soil) |
Methanogenic Bacteria (cells/g dry soil) |
Methanogenesis (µmol CH4/g dry soil/day) |
9-I |
3 x 10^1 |
3 x 10^1 |
1.8 |
9-II |
1.5 x 10^5 |
10^3 |
1.5 |
TLC Systems and Rf-Values
No. |
Solvent* |
Rf Value |
|
Test Material |
2-methyl-4-chlorophenol |
||
A |
Chloroform cyclohexane acetic-acid= 80 20 10 |
0.7 |
0.7 |
B |
Benzene dioxan acetic acid= 90 25 4 |
0.5 |
0.8 |
F |
Acetonitrile water aq. ammonia (25 %) = 80 18 2 |
0.5 |
0.9 |
The adsorbent was silica gel Merck No. 5715 in all cases.
* All values are v/v without chamber saturation.
Data on the Water During the Experiment
Period after application [d] |
Krempe |
Ohlau |
||||
pH |
O2 Conc.** [mg/L] |
Temp [°C] |
pH |
O2 Conc. [mg/L] |
Temp [°C] |
|
0 |
7.4 |
6.6 |
21.5 |
6.6 |
7.0 |
21.5 |
3 |
7.7 |
7.7 |
21.7 |
6.6 |
7.1 |
21.7 |
7 |
7.3 |
3.8 |
21.8 |
6.9 |
7.3 |
21.8 |
14 |
7.9 |
7. |
21.9 |
7.0 |
8.1 |
21.9 |
30 |
7.7 |
8.0 |
21.2 |
6.4 |
8.7 |
21.2 |
60 |
7.3 |
8.3 |
22.1 |
5.8 |
8.5 |
21.1 |
91/ 92* |
7.6 |
8.5 |
23.1 |
6.1 |
8.2 |
23.5 |
*The last sampling was on day 91 for the systems Krempe and on day 92 for the system Ohlau.
**At 22 °Cthe saturation is equivalent to 9 mg o2 per L.
Microbiological Data on the Water in the Control Systems
Day After Application |
Total Germ [count/mL]** |
|
Krempe |
Ohlau |
|
-19* |
7300 |
240 · 10^3 |
7500 |
360 · 10^3 |
|
0 |
920 |
5100 |
440 |
2200 |
|
540 |
|
|
30 |
240 |
1140 |
1510 |
910 |
|
2400 |
|
|
60 |
780 |
2190 |
87 |
|
|
91 |
183 |
158 |
204 |
208 |
|
380 |
|
* Day of collecting the water-sediment samples from the respective sources
** The individual results of replicate sample are shown.
Radioactivity Distribution and Balance During the Degradation of the Test Material
Test Period [d] |
Amount of Radioactivity [%]* |
||||
Sediment |
Water |
Volatiles |
∑ (Balance) |
||
CO2 |
Others |
||||
System: Krempe |
|||||
0 |
6.2 |
99.2 |
ND |
ND |
105.4 |
3 |
13.6 |
85.1 |
0.3 |
0.4 |
99.3 |
7 |
16.3 |
83.9 |
1.0 |
0.5 |
101.7 |
14 |
16.5 |
83.0 |
1.5 |
0.4 |
101.4 |
30 |
26.5 |
16.2 |
47.9 |
0.2 |
90.8 |
60 |
19.9 |
3.3 |
76.1 |
< 0.1 |
99.3 |
91 |
18.7 |
1.6 |
82.9 |
< 0.1 |
13.2 |
System: Ohlau |
|||||
0 |
5.7 |
98.5 |
ND |
ND |
104.2 |
3 |
14.0 |
85.3 |
1.0 |
0.4 |
100.7 |
7 |
14.5 |
84.7 |
1.8 |
0.3 |
101.3 |
14 |
17.5 |
81.3 |
3.1 |
0.4 |
102.3 |
30 |
28.0 |
6.6 |
58.0 |
0.3 |
92.9 |
60 |
16.4 |
6.2 |
67.9 |
0.1 |
90.6 |
92 |
28.9 |
3.0 |
79.7 |
< 0.1 |
111.6 |
*Percentage of radioactivity related to the amount originally applied to the system
ND:Not determined
Amounts of Parent Compound During the Degradation of the Test Material
Test Period [d] |
Amount of Parent Compound [%]* in Extracts |
||
Sediment |
Water |
∑ |
|
System: Krempe |
|||
0 |
5.2 |
92.3 |
97.5 |
3 |
9.0 |
75.1 |
84.1 |
7 |
11.0 |
81.0 |
92.0 |
14 |
10.5 |
75.7 |
86.2 |
30 |
0.6 |
0.6 |
1.2 |
60 |
0.2 |
0.4 |
0.6 |
91 |
< 0.1 |
< 0.4 |
< 0.4 |
System: Ohlau |
|||
0 |
5.1 |
94.5 |
99.9 |
3 |
9.1 |
71.7 |
80.8 |
7 |
7.8 |
79.7 |
87.5 |
14 |
8.6 |
74.3 |
82.9 |
30 |
1.1 |
0.7 |
1.8 |
60 |
0.2 |
< 0.3 |
< 0.5 |
92 |
< 0.1 |
< 0.3 |
< 0.5 |
*Percentage of radioactivity found at the Rf value for parent compound, related to the amount originally applied to the system
Comprehensive Table for the Degradation of Test Material
Test Period [d] |
Amount of Radioactivity [%]* Found |
|||||
Parent Compound |
Degradates** |
Unextract. [Sediment] |
Polar [Water] |
CO2 |
∑ [Balance] |
|
System: Krempe |
||||||
0 |
97.5 |
5.4 |
0.7 |
2.0 |
ND |
105.6 |
3 |
84.1 |
2.9 |
3.2 |
2.0 |
0.6 |
92.8 |
7 |
92.0 |
3.2 |
4.0 |
2.1 |
1.1 |
102.4 |
14 |
86.2 |
2.3 |
4.8 |
2.9 |
2.3 |
98.4 |
30 |
1.2 |
4.7 |
22.2 |
2.0 |
61.0 |
91.1 |
60 |
0.6 |
2.9 |
17.4 |
1.2 |
77.2 |
99.3 |
91 |
< 0.4 |
< 2.6 |
16.9 |
0.6 |
84.2 |
101.7† |
System: Ohlau |
||||||
0 |
99.9 |
2.9 |
0.4 |
1.8 |
ND |
105.0 |
3 |
80.8 |
7.6 |
2.9 |
2.3 |
1.0 |
94.6 |
7 |
87.5 |
6.5 |
4.8 |
2.2 |
1.8 |
102.8 |
14 |
82.9 |
2.5 |
3.6 |
2.6 |
3.2 |
98.8 |
30 |
1.8 |
5.0 |
23.4 |
3.1 |
58.2 |
91.5 |
60 |
< 0.5‡ |
< 5.4‡ |
10.7 |
4.7 |
68.2 |
89.5 |
92 |
< 0.3 |
< 3.2 |
25.9 |
2.3 |
79.9 |
108.1⁑ |
*Percentage of radioactivity related to the amount originally applied to the system = 100%
** Consists of 1 to 5 peaks in radio-TLC
ND: Not determined
†This figure of 101.7%is the sum of unextractable, polar and CO2 only.
‡The extract from the sediment contained 0.2% parent compound and 5.4% degradates, whereas the total amount of radioactivity in the extract from the water was 0.3 only which was not investigated via radio-TLC.
⁑ This figure of 108.1% is the sum of unextractable, polar and co2 only.
Description of key information
Key study: Roohi & Perry (2015) - Read across (MCPP-P)
Under the conditions of this water/ sediment (OECD 308 guideline) study, [14C]-test material in natural water/sediment systems was shown to degrade ultimately to carbon dioxide and unextractable sediment bound residues. DT50 and DT90 values in the water phase were 72.5 - 171 days and 91.9 - 567 days. Whole system DT50 and DT90 values were 83.2 - 244 days and 96.7 and 810 days, respectively. The redox potentials and oxygen contents showed that the water phases remained aerobic throughout the study whilst the sediments were anaerobic and remained in this state for the duration of the study.
Key study: Traub (2014) - Read across (MCPP-P)
Under the conditions of this aerobic mineralisation (OECD 309) guideline study MCPP-P was shown to be essentially stable, with DT50 values for [14C]test material extrapolated far beyond the 58 day study duration and determined to be 2 501 days (10 μg/L) and 9 621 days (100 μg/L) in the water phases. The DT90 values for [14C]test material were determined to 8 308 days (10 μg/L) and 31 960 days (100 μg/L) in the water phases. Therefore, according to the results of the study, the test material is persistent.
Supporting study: Harrison et al. (2003)
The laboratory microcosms confirmed the processes contributing to variations in enantiomeric fraction of test material down gradient from a landfill in a limestone aquifer.
They revealed that the test material does not degrade in methanogenic/sulphate-reducing and iron-reducing conditions. In nitrate-reducing conditions R-isomer degrades but S-isomer does not. In aerobic conditions S-isomer degrades at a faster rate than R-isomer. There was no indication of enantiomeric inversion.
It was also possible to show that anaerobic transformation proceeded by initial cleavage of the ether linkage leading to formation of the transient metabolite 4-CMP. Only when all R-isomer had been converted to 4-CMP did the metabolite itself begin to biodegrade anaerobically.
Supporting study: Williams et al. (2003)
This study has shown that systematic change in the enantiomeric fraction of racemic test material deposited in the landfills at Helpston is a useful indicator of in situ processes and provides insight into the relative behaviour of the enantiomers under different redox conditions. Test material behaviour inferred from field data is supported by the results of microcosm experiments conducted under aerobic and anaerobic conditions. No degradation occurred in methanogenic, sulphate-reducing or iron-reducing microcosms. In nitratereducing microcosms, S-isomer did not degrade, but R-isomer degraded with zero-order kinetics at 0.65 mg/L/day. The associated buildup of 4-chloro-2-methylphenol indicated cleavage of the propionic moiety at the ether linkage, and this metabolite only degraded after the R-isomer had disappeared. In aerobic microcosms, the S-isomer degraded faster than the R-isomer both following zero-order kinetics 1.32 and 1.9 mg/L/day. The nature of the microbial community responsible for test material degradation is currently under study.
Supporting study: Larsen et al. (2001)
The test material was readily mineralised and was mineralised under both aerobic and anaerobic conditions. Under aerobic conditions (S0 slurries) mineralisation started immediately, and 36 % of the test material had mineralised by the end of the experiment, 473 days later. Under anaerobic conditions (S5 slurries), in contrast, only 8 % of the test material mineralised during the course of the experiment. Similarly low rates of mineralisation were obtained under both sulfate-reducing (S9-I: 10 %) and methanogenic conditions (S9-II; 13 %).
Supporting Study: Bieber & Krohn (1991) - Read across (MCPP-P)
Under the conditions of the study the degradation of the test material proceeded rather rapidly in both systems. The main degradation product was carbon dioxide, which after three months comprised more than 80 % of the radioactivity originally applied to the system. Within this time the amount of parent compound decreased to less than 0.4 %. At the end of the test period three months after application approximately 4 to 8 % of the radioactivity were found in humic acids in the systems, another 3 to 4 % in fulvic acids. Ca. 11 % were retained in one sediment, ca. 8 % only in the other.
A disappearance time (DT) 50 of 20 to 21 days was found, the DT 90 was 28 days. This low DT 90 value may be explained by the exponential growth of the microbial degraders after a lack phase in the beginning of the experiment.
Supporting Study: Cooper & Unsworth (1996) - Read across (MCPP-P)
Under the conditions of the study the test material is readily degraded in the aerobic aquatic systems and is therefore unlikely to persist in the aquatic environment.
Supporting Study: Buser & Müller (1998) - Read across (MCPP-P)
Under the conditions of the study the concentrations of the test material were reduced during the 50 - 129 d of incubation in both experiments. The data fitted approximate first-order kinetics without a lag phase.
The data indicate the test material is relatively stable in the aquatic environment whereas it degrades relatively quickly in soil (half-life, 7 - 22 d). The data for the test material show an approximately constant concentration (55 ng/L; lag phase) up to 21 d, followed by a gradual decrease to ≈ 11 ng/L at 89 d, the largest decrease occurring between 21 and 63 d.
Supporting Study: Hazlerigg & Garrett (2014) - Read across (MCPP-P)
The data did not allow a firm relationship to be built-up between the partitioning of test material to the sediment and degradation in the water phase in a two-compartment analysis. This was primarily a function of limited back-transfer from the sediment back into the water column.
Key value for chemical safety assessment
- Half-life in freshwater:
- 111.3 d
- at the temperature of:
- 20 °C
Whole System
- Half-life in whole system:
- 142.5 d
- at the temperature of:
- 20 °C
- Type of system:
- fresh water and sediment
Additional information
Two key studies (Klimisch score: 1) are available to describe the fate and behaviour of mecoprop in the aquatic environment. The studies of Roohi & Perry (2015) and Traub (2014) investigated the route and rate of degradation MCPP-P acid in two water/ sediment systems (OECD 308 guideline) and a single natural aerobic water column (OECD 309 guideline), respectively. Both studies were performed in such a way as to maintain aerobic conditions in water phases; the redox potentials and oxygen contents showed that the water phases remained aerobic throughout the study whilst the sediments in the water/ sediment study were (as is typical for water/ sediment studies) anaerobic and remained in this state for the duration of the study. Considering that partitioning from water to sediment was slow and that the majority of the radioactivity remained in the water phase of both water/ sediment systems, the majority of the test item was subjected to aerobic, rather than anaerobic, conditions during both studies.
Seven supporting studies are available; three performed with racemic mecoprop as the test item. Collectively the supporting studies demonstrate that under typical anaerobic conditions both the R and S isomers of mecoprop are stable or degrade very slowly. Only in the specific case of nitrate-reducing conditions does the R-isomer degrade more rapidly than the S-isomer. In aerobic conditions, the S-isomer degrades at a faster rate than R-isomer. Therefore, under aerobic conditions the R-isomer degrades more slowly than the S-, and rate of degradation studies performed with MCPP-P (R-isomer) provide conservative degradation rates for racemic mecoprop. Thus, considering the very close structural similarity between the source and target substances (as justified in the read-across report that is attached to section 13 of the dossier) and the likely conservative nature of aquatic aerobic degradation studies in relation to racemic mecoprop, results from the studies performed with mecoprop-p can be reliably extrapolated to mecoprop. The two studies of Roohi & Perry (2015) and Traub (2014), performed with MCPP-P acid provide conservative degradation rates for racemic mecoprop and are suitable to support the mecoprop dossier.
The water/sediment study of Roohi & Perry (2015) demonstrated a geometric mean whole system DT50 of 142.5 d and a geometric mean water phase dissipation DT50 of 111.3 d for MCPP-P from the two systems. The aerobic mineralisation study of Traub (2014) demonstrated that MCPP-P degraded only very slowly in the water column (actual degradation rates uncertain due to extrapolation significantly beyond the study duration).
Key study: Roohi & Perry (2015) - Read across (MCPP-P)
The route and rate of degradation of the test material in two water/sediment systems was investigated in accordance with the standardised guideline OECD 308 under GLP conditions. The study was awarded a reliability score of 1 in accordance with the criteria set forth by Klimisch et al. (1997).
The route and rate of degradation of [14C]-test material has been investigated under aerobic conditions at 20 ± 2 °C in two contrasting water sediment systems in the dark. The water sediment systems were Calwich Abbey [Staffordshire, UK, silt loam sediment, pH 7.2, 5.0 % OC] and Swiss Lake [Derbyshire, UK, loamy sand sediment, pH 6.6, 0.7 % OC]. The systems were incubated in glass flasks containing sediment and associated water at a ratio of approximately 1:3 (v/v). Throughout the experiment the flasks were maintained in the dark at 20 ± 2 °C whilst attached to an incubation system allowing air to be bubbled through the surface water and then through a system for trapping volatile degradates. The water/sediment systems were incubated for 7 days prior to application of [14C]-test material to allow the systems to equilibrate. The redox potential of the sediment and water in control flasks was measured at regular intervals during the incubation. The pH and dissolved oxygen content of the water were also measured.
[14C]-test material was applied to the water surface of individual water sediment systems at a target application rate equivalent to an initial concentration of 0.138 mg/L in the water phase, equivalent to the direct overspray from a field application at a rate of 1 380 g/ha to a water body of 100 cm depth. The treated sediment systems were incubated for up to 98 days.
At zero time (immediately after treatment) and at intervals of 7, 14, 29, 56, 81 and 98 days after treatment duplicate flasks and their corresponding traps were removed from the incubation system. The water was carefully decanted, and the sediment was extracted once with acetonitrile, then two more times with acetonitrile: water (80:20 v/v) at ambient temperature. Extracted sediment samples were air-dried (for the early time points), ground to a fine powder and the residual radioactivity quantified by combustion. For the later time points (day 56 onwards), in order to avoid possible loss of any volatile material during drying, the sediments were not dried before combustion. The radioactivity in the water, the sediment extracts and the volatile traps was quantified by liquid scintillation counting (LSC).
Following extraction, the water phase and the sediment extracts were analysed directly by reverse phase high performance liquid chromatography (HPLC).
The overall recovery of radioactivity was good throughout the study with mean values of 96.9 % of applied radioactivity (AR) for the Calwich Abbey system and 99.7 % AR for the Swiss Lake system. Recoveries for individual flasks ranged from 93.1 to 102.0 % AR for the Calwich Abbey system and from 95.3 to 101.7 % AR for the Swiss Lake system.
In the Calwich Abbey system the levels of radioactivity in the water declined steadily from 101.3 % AR to 48.2 % AR at day 81. After this initial lag phase, however, the rate of decline from the water accelerated rapidly, reaching 4.1 % AR at day 98. The total extractable radioactivity in the sediment rose from 0.0 % AR at time zero to a maximum of 23.0 % at day 56 before declining to 6.6 % at the end of the study at day 98. The unextracted radioactivity rose from 0.0 % at time zero to 32.3 % at day 98. The presence of an initial metabolic lag phase was also evident in the evolution of CO2. Over the first 56 days only 4.9 % CO2 was released, however, this had increased to 14.8 % AR by day 81 and reached 50.1 % AR by day 98.
In the Swiss Lake system there was a slower transfer of the applied radioactivity from the water to the sediment, when compared with Calwich Abbey system, and thus resulting in a much greater percentage of applied radioactivity remaining in the water at the end of the incubation period. The radioactivity in the water declined from 101.1 % AR at time zero to 62.7 % AR by day 98. The total radioactivity in the sediment increased from 0.0 % at time zero to 21.8 % by day 98, with the extractable portion reaching a maximum of 12.8 % AR at day 56 and the unextractable reaching a maximum of 10.4 % AR at day 98. The degree of mineralisation to CO2 was less than in the Calwich Abbey system accounting for 13.5 % AR by day 98.
The chromatographic results showed that in both the Calwich Abbey and Swiss Lake systems, the applied test material partitioned into the sediment and was degraded to form minor metabolites, none exceeding >5.0 % AR.
In the total Calwich Abbey system, the applied test material declined from 101.3 % AR at time zero to 66.8 % AR at day 81. After day 81 degradation accelerated rapidly such that only 8.7 % AR remained as test material by day 98. No significant metabolites (i.e. >10 % AR, >5 % AR at consecutive sampling intervals or >5 % AR without decline at end of study) were detected. In the water phase, the applied test material declined from 101.3 % AR at time zero to 47.5 % AR at day 81 and to 3.7 % AR at day 98. In the sediment extracts, the test material reached a maximum level of 22.1 % AR at day 56 and subsequently declined to 5.0 % AR by day 98.
In the total Swiss Lake system, the applied test material declined from 101.1 % AR at time zero to 73.4 % AR at day 98. No significant metabolites (i.e. >10 % AR, >5 % AR at consecutive sampling intervals or >5 % AR without decline at end of study) were detected. In the water phase, the applied test material declined from 101.1 % AR at time zero to 62.1 % AR at day 98. In the sediment extracts, the test material reached a maximum level of 13.0 % AR at day 14 and subsequently declined slightly to 11.4 % AR by the end of the study at day 98.
The dissipation of the test material from the water phase and degradation in the total system was evaluated according to the FOCUS guidance document on degradation kinetics using the most appropriate model for the best fit to the data set. In the Calwich Abbey system, with the data exhibiting an initial lag-phase of moderate degradation followed by rapid decline, the Hockey-stick (HS) model was selected as best-fit to the data for the water phase and for the total system. In the Swiss Lake system the SFO model was selected as best-fit for the degradation in the water and for the total system.
Under aerobic conditions in the Calwich Abbey system, [14C]-test material dissipated rapidly from the water phase after an initial lag phase with a best-fit overall DT50 value of 72.5 days.
Dissipation from the water phase was slower in the Swiss Lake system with a DT50 of 171 days. The degradation in the total water/sediment systems again showed differences in the two systems, with best fit DT50 values of 83.2 and 244 days for the Calwich Abbey and Swiss Lake systems, respectively.
In conclusion, under the conditions of this study, [14C]-test material in natural water/sediment systems was shown to degrade ultimately to carbon dioxide and unextractable sediment bound residues.
Key study: Traub (2014) - Read across (MCPP-P)
The rate of degradation of the test material, and the number and quantity of formed metabolites, were investigated in a study which was conducted in accordance with the standardised guideline OECD 309, under GLP conditions.The study was awarded a reliability score of 1 in accordance with the criteria set forth by Klimisch et al. (1997).
The study was performed under aerobic conditions in the dark using natural aerobic surface water taken from a large water body. To be able to determine the degradation rate and to follow the transformation products two different application rates (10 and 100 μg/L) of radio labelled test material were applied. Assuming a specific activity of 8.24 MBq/mg this corresponds to a spiked radioactivity of about 0.04 and 0.41 MBq per vessel, respectively.
Water taken from Rhineland-Palatinate (67 374 Hanhofen, Germany, 49°31’N, 08°32’O) was used as the test system. This water had a dissolved organic carbon content of 8.6 mg/L and a BOD5 of < 3 mg/L.
The water system was incubated in the dark at 20 ± 2°C under constant bubbling of air through the water. The incubation period after treatment was 58 days. Organic volatiles and carbon dioxide were trapped.
Duplicate samples were taken for analysis at specified intervals up to 58 days after application. The radioactivity was quantified by liquid scintillation counting and characterised by normal phase thin layer chromatography. Reversed phase thin layer chromatography was used for confirmation of metabolites in selected samples.
The mean recoveries of both test concentrations were within the range 97 to 101 % of the applied radioactivity (AR).
The mineralisation rate was negligible for both tested concentrations. Only amounts < 5 % AR were detected as CO2. The amount of solved CO2 in the sodium hydroxide traps was negligible. Organic volatiles were detected < 1 % AR. For both concentrations no metabolites were formed during the incubation period in the water system.
The test system was validated by the help of the reference material sodium benzoate. After 13 days 82 - 87 % of the reference material was mineralised.
Under the conditions of this study, the DT50 values for [14C]test material were determined to be 2501 days (10 μg/L) and 9621 days (100 μg/L) in the water phases. The DT90 values for [14C]test material were determined to be 8308 days (10 μg/L) and 31 960 days (100 μg/L) in the water phases. Therefore, according to the results of the study, the test material is persistent.
Supporting study: Harrison et al. (2003)
The natural attenuation of test material was studied by determining changes in enantiomeric fraction in different redox environments down gradient from a landfill in the Lincolnshire limestone. Such changes could be due to differential metabolism of the enantiomers, or enantiomeric inversion. In order to confirm the processes occurring in the field, microcosm experiments were undertaken using limestone acclimatised in different redox zones. The study was awarded a reliability score of 2 in accordance with the criteria set forth by Klimisch et al. (1997).
No biodegradation was observed in the methanogenic, sulphate-reducing or iron-reducing microcosms. In the nitrate-reducing microcosm S-isomer did not degrade but R-isomer degraded with zero order kinetics at 0.65 mg/L/day to produce a stoichiometric equivalent amount of 4-chloro-2-methylphenol. Only when R-isomer disappeared did biodegradation of 4-CMP commence. This suggested that 4-CMP was a transient metabolite formed by cleavage of the ether linkage between the aromatic and propionic acid moieties of R-isomer.
The addition of nitrate to dormant iron-reducing microcosms devoid of nitrate stimulated anaerobic degradation of R-isomer after a lag period of about 20 days and was associated with the production of 4-chloro-2-methylphenol. Nitrate addition to sulphate-reducing/methanogenic microcosms did not stimulate test material degradation. However, the added nitrate was completely utilised in oxidising sulphide to sulphate. There was no evidence for enantiomeric inversion.
The study reveals new evidence for fast enantioselective degradation of R-isomer under nitrate-reducing conditions.
Supporting study: Williams et al. (2003)
Test material degradation in different redox environments was tested within a Lincolnshire Limestone aquifer. The objective of the study was to assess whether changes in the enantiomeric fraction can be used to indicate natural attenuation in the field. The study was awarded a reliability score of 2 in accordance with the criteria set forth by Klimisch et al. (1997). The study involved sampling groundwater and relating systematic changes in enantiomeric fraction to different redox environments down gradient of the landfill. Laboratory microcosms were set up with groundwater and limestone acclimatised with the in situ microbial consortium to confirm the behaviour of the test material enantiomers under controlled conditions.
This study has shown that systematic change in the enantiomeric fraction of racemic test material deposited in the landfills at Helpston is a useful indicator of in situ processes and provides insight into the relative behaviour of the enantiomers under different redox conditions. Test material behaviour inferred from field data is supported by the results of microcosm experiments conducted under aerobic and anaerobic conditions. No degradation occurred in methanogenic, sulphate-reducing or iron-reducing microcosms. In nitratereducing microcosms, S-isomer did not degrade, but R-isomer degraded with zero-order kinetics at 0.65 mg/L/day. The associated buildup of 4-chloro-2-methylphenol indicated cleavage of the propionic moiety at the ether linkage, and this metabolite only degraded after the R-isomer had disappeared. In aerobic microcosms, the S-isomer degraded faster than the R-isomer both following zero-order kinetics 1.32 and 1.9 mg/L/day. The nature of the microbial community responsible for test material degradation is currently under study.
Supporting study: Larsen et al. (2001)
The potential of a riparian fen to mineralise herbicides that could leach from an adjacent catchment area was investigated. The study was awarded a reliability score of 2 in accordance with the criteria set forth by Klimisch et al. (1997). Slurries were prepared from sediment and ground water collected from different parts of a wetland representing different redox conditions. The slurries were amended with O2, NO3^-, SO4^2-, and CO2, or CO2 alone as electron acceptors to simulate the in situ conditions and their ability to mineralise the herbicide test material. In addition, the abundance of bacteria able to utilise O2, NO3^-, SO4^2- + CO2 and CO2 as electron acceptors was investigated along with the O2–reducing and methanogenic potential of the sediment. The study was awarded a reliability score of 2 in accordance with the criteria set forth by Klimisch et al. (1997).
Study findings revealed that aerobic bacterial abundance was one order of magnitude greater in the aerobic sediment (S0: 5.8 x 10^6 CFU) than in the denitrifying sediment (S5: 6.4 x 10^5 CFU). The abundance of denitrifying bacteria was lower than that of aerobic bacteria under both aerobic (S0) and denitrifying (S5) conditions. The number of bacteria using the prevalent electron acceptors was lower in the sulfate-reducing (S9-I) and methanogenic (S9-II) sediment than in the aerobic (S0) and denitrifying (S5) sediment. Both sulfate-reducing and methanogenic bacteria were more abundant in the methanogenic sediment (S9-II) than in the sulfate-reducing sediment (S9-I). However, the potential rates of methanogenesis did not differ significantly between these two sediments.
Under the conditions of the study, the test material was readily mineralised and was mineralised under both aerobic and anaerobic conditions. Under aerobic conditions (S0 slurries) mineralisation started immediately, and 36 % of the test material had mineralised by the end of the experiment, 473 days later. Under anaerobic conditions (S5 slurries), in contrast, only 8 % of the test material mineralised during the course of the experiment. Similarly low rates of mineralisation were obtained under both sulfate-reducing (S9-I: 10 %) and methanogenic conditions (S9-II; 13 %). Moreover, mineralisation of the test material in both S9-I and S9-II slurries was independent of the presence of added sulfate since the omission of sulfate from S9-I slurries and the addition of sulfate to S9-II slurries did not significantly affect the mineralisation of the test material. Also, there was no significant difference between the amounts of accumulated 14CO2 from test material in anaerobic slurries amended with nitrate and anaerobic slurries with sulfate or without additional electron acceptor.
Supporting Study: Bieber & Krohn (1991) - Read across (MCPP-P)
The degradation of the test material in sediment/water was assessed according to Pesticide Assessment Guidelines Subdivision N, § 162-4 and in compliance with GLP. The study was awarded a reliability score of 2 in accordance with the criteria set forth by Klimisch et al. (1997).
The degradation of the test substance [14 C]-test material, being labelled in the aromatic ring was investigated in two different water/sediment systems under aerobic conditions over a period of three months at 22 °C. 1.88 mg test material was applied per kg of the system.
After an incubation time of approximately two weeks, the degradation of the test material proceeded rather rapidly in both systems. The main degradation product was carbon dioxide, which after three months comprised more than 80 % of the radioactivity originally applied to the system. Within this time the amount of parent compound decreased to less than 0.4 %.
At the end of the test period three months after application approximately 4 to 8 % of the radioactivity were found in humic acids in the systems, another 3 to 4 % in fulvic acids. Ca. 11 % were retained in one sediment, ca. 8 % only in the other.
For both systems a disappearance time (DT) 50 of 20 to 21 days was found, the DT 90 was 28 days. This low DT 90 value may be explained by the exponential growth of the microbial degraders after a lack phase in the beginning of the experiment.
Supporting Study: Cooper & Unsworth (1996) - Read across (MCPP-P)
The rate of test material degradation in two aquatic sediment systems was assessed according to BBA) Guidelines, Part IV, Section 5-1 and in compliance with GLP. The study was awarded a reliability score of 2 in accordance with the criteria set forth by Klimisch et al. (1997).
The degradation of [14C]-test material, applied at a rate equivalent to 1.123 kg ai ha^-1, has been studied in two different aquatic sediment systems over a period of 100 days at 20 °C. The two sediments differed in that the Manningtree sediment had higher organic carbon and nitrogen content, whilst the Ongar system was more alkaline with a much higher cation exchange capacity.
The incubation was performed in glass flasks (approximately 7.5 cm internal diameter), containing sediment to an approximate depth of 2.5 cm covered with associated water to an approximate depth of 5 cm above the sediment. The units were maintained in the dark at 20 °C ± 2 °C. The water/sediment systems were incubated for approximately 6 weeks to enable acclimatisation, prior to [14C]-test material application to the surface water.
Moistened carbon dioxide-free air was supplied under positive pressure through the water in each unit and the effluent air passed through an ethylene glycol and two 2M potassium hydroxide traps, to trap any volatile products and liberated carbon dioxide (14CO2) respectively.
A good recovery of radioactivity was obtained for both systems with an overall mean recovery of 110.0 % (range 114.0 - 103.7 %) for system 96/03 (Manningtree). And 105. 5% (range 112.6 - 87.4 %) for system 96/04 (Ongar).
There was a steady transfer of the radioactivity from the water to the sediment in both systems, commencing at 111.29 % in the water phase at zero time and declining to 15.43 % (Manningtree system) and 1.80 % (Ongar system) after 100 days.
Extractable residues rose from 0 % at the first time-point (no extraction was made) to a maximum of 13.48 % at 14 days (Manningtree system). The Ongar system reached a maximum extracted residue level of 6.64 % after 14 days. Unextactable residues in both systems fluctuated with time from 0.77 % (Manningtree system) and 2.80 % (Ongar system) at time zero to 27.98 % (Manningtree system) at 100 days and 39.67 % (Ongar system) at 61 days.
Volatiles characterised as carbon dioxide accounted for 55 % (range 79 - 31 %) (Manningtree system) and 58 % (range 42 - 73 %) (Ongar system) of the applied dose after 100 days. Volatile materials, trapped in ethylene glycol, accounted for a negligible fraction (< 0.1 %) of the applied dose after 100 days. The test material was found to be the main component present in both the water and sediment phases. In addition significant mineralisation to carbon dioxide occurred. Some minor metabolites were observed in both the sediment and water phases, none of which exceeded 10 % of applied radioactivity in the total system.
Manningtree sediment: Water phase
DT50 = 49.23 days
DT90 = 155.47 days
Manningtree sediment: Complete system
DT50 = 60.66 days
DT90 = 175.23 days
Ongar sediment: Water phase
DT50 = 24.25 days
DT90 = 33.27 days
Ongar sediment: Complete phase
DT50 = 22.97 days
DT90 = 26.42 days
Under the conditions of the study the test material is readily degraded in the aerobic aquatic systems and is therefore unlikely to persist in the aquatic environment.
Supporting Study: Buser & Müller (1998) - Read across (MCPP-P)
The degradation of the test material in surface water was determined in surface water collected from five lakes and two rivers situated in the central and eastern region of Switzerland. The study was awarded a reliability score of 2 in accordance with the criteria set forth by Klimisch et al. (1997).
The water from Sempachersee was incubated in 1 L green glass bottles and incubated for up to 129 d at room temperature (20 - 23 °C). Periodically, one of the bottles was removed from storage, and the water was analysed.
The water from the river Aabach was incubated in one batch in a 2.5-L clear glass bottle for up to 50 d at room temperature while being stirred with a Teflon bar. Periodically, 0.25 L subsamples were removed and analysed. From the amounts of oxygen dissolved and the relatively small amounts of dissolved organic matter, it can be assumed that the incubation conditions in both experiments were aerobic.
There was some algal growth observed in the clear glass bottle.
Additionally, incubation experiments were carried out with fortified water, using water from Sempachersee and the river Aabach. One 2.5-L batch of each were fortified at 50 - 100 ng/L with the test material.
The waters were incubated at room temperature in amber bottles for up to 89 d. Periodically, 0.25 L subsamples were removed and analysed. The fortifications were made by adding 1.0 mL of a 250 ng/ mL solution of the compounds in distilled water, prepared from stock solutions of the compounds in methanol.
Extraction from the acidified (pH ≈ 2) water was effected with a reusable small column containing a macroporous polystyrene adsorbent, and the analytes were eluted and recovered. After methylation with diazomethane, the extracts were concentrated (≈0.5 mL in diethyl ether) and passed through a small silica column (0.7 g of silica gel 60, deactivated with 5 % water; 5 mm i.d. Pasteur pipet) topped with 10 mm of sodium sulfate. The analytes (as methyl esters) were eluted with 10 mL ofn-hexane-methylene chloride (1:1). After careful concentration and dilution to 100 - 200μL withn-hexane, 2 μL aliquots were used for analysis by high-resolution gas chromatography/mass spectrometry (HRGC/MS).
Under the conditions of the study the concentrations of the test material were reduced during the 50 - 129 d of incubation in both experiments. The data fitted approximate first-order kinetics without a lag phase.
The data indicate the test material is relatively stable in the aquatic environment whereas it degrades relatively quickly in soil (half-life, 7 - 22 d). The data for the test material show an approximately constant concentration (55 ng/L; lag phase) up to 21 d, followed by a gradual decrease to ≈ 11 ng/L at 89 d, the largest decrease occurring between 21 and 63 d.
Supporting Study: Hazlerigg & Garrett (2014) - Read across (MCPP-P)
The report covers the re-analysis of data from a previous study (Cooper & Unsworth, 1996) of the behaviour of the test material in water-sediment systems, according to the latest guidelines, in order to provide the most appropriate data for triggers for further studies and for inputs into environmental models of pesticide fate, specifically for use in FOCUS STEPS 1-4 for surface water modelling (FOCUS 2001, 2012). The study was awarded a reliability score of 4 in accordance with the criteria set forth by Klimisch et al. (1997).
FOCUS (2006) guidance on kinetics was used to evaluate two water-sediment datasets, Manningtree and Ongar. Two ‘Levels’ of analysis were used: Level P1, looking at a single compartment model and Level P2, looking at a two-compartment model with transfer between the water and sediment and vice versa.
The kinetic analysis conducted shows some differences to that originally performed. The values for Manningtree for both water and the whole system are similar to those previously reported. However, the values for Ongar for both water and the whole system are higher than those originally reported. The cause of the differences is likely due to the preparation of the data for analysis (exclusion of outliers) and the use of an SFO kinetics model rather than the three-compartment decay model used in the original analysis. This results in conservative degradation kinetic values for use in environmental fate modelling.
The data did not allow a firm relationship to be built-up between the partitioning of test material to the sediment and degradation in the water phase in a two-compartment analysis. This was primarily a function of limited back-transfer from the sediment back into the water column. In practice, this means that whole system DegT50 must be used in FOCUS STEP 3 modelling for one compartment (water) and the default DT50 of 1000 days used for the other compartment (sediment). This allocation is driven by the KOC of the test material as recommended by FOCUS (2012) guidance.
Information on Registered Substances comes from registration dossiers which have been assigned a registration number. The assignment of a registration number does however not guarantee that the information in the dossier is correct or that the dossier is compliant with Regulation (EC) No 1907/2006 (the REACH Regulation). This information has not been reviewed or verified by the Agency or any other authority. The content is subject to change without prior notice.
Reproduction or further distribution of this information may be subject to copyright protection. Use of the information without obtaining the permission from the owner(s) of the respective information might violate the rights of the owner.
