Registration Dossier
Registration Dossier
Data platform availability banner - registered substances factsheets
Please be aware that this old REACH registration data factsheet is no longer maintained; it remains frozen as of 19th May 2023.
The new ECHA CHEM database has been released by ECHA, and it now contains all REACH registration data. There are more details on the transition of ECHA's published data to ECHA CHEM here.
Diss Factsheets
Use of this information is subject to copyright laws and may require the permission of the owner of the information, as described in the ECHA Legal Notice.
EC number: 269-927-8 | CAS number: 68391-08-2
- Life Cycle description
- Uses advised against
- Endpoint summary
- Appearance / physical state / colour
- Melting point / freezing point
- Boiling point
- Density
- Particle size distribution (Granulometry)
- Vapour pressure
- Partition coefficient
- Water solubility
- Solubility in organic solvents / fat solubility
- Surface tension
- Flash point
- Auto flammability
- Flammability
- Explosiveness
- Oxidising properties
- Oxidation reduction potential
- Stability in organic solvents and identity of relevant degradation products
- Storage stability and reactivity towards container material
- Stability: thermal, sunlight, metals
- pH
- Dissociation constant
- Viscosity
- Additional physico-chemical information
- Additional physico-chemical properties of nanomaterials
- Nanomaterial agglomeration / aggregation
- Nanomaterial crystalline phase
- Nanomaterial crystallite and grain size
- Nanomaterial aspect ratio / shape
- Nanomaterial specific surface area
- Nanomaterial Zeta potential
- Nanomaterial surface chemistry
- Nanomaterial dustiness
- Nanomaterial porosity
- Nanomaterial pour density
- Nanomaterial photocatalytic activity
- Nanomaterial radical formation potential
- Nanomaterial catalytic activity
- Endpoint summary
- Stability
- Biodegradation
- Bioaccumulation
- Transport and distribution
- Environmental data
- Additional information on environmental fate and behaviour
- Ecotoxicological Summary
- Aquatic toxicity
- Endpoint summary
- Short-term toxicity to fish
- Long-term toxicity to fish
- Short-term toxicity to aquatic invertebrates
- Long-term toxicity to aquatic invertebrates
- Toxicity to aquatic algae and cyanobacteria
- Toxicity to aquatic plants other than algae
- Toxicity to microorganisms
- Endocrine disrupter testing in aquatic vertebrates – in vivo
- Toxicity to other aquatic organisms
- Sediment toxicity
- Terrestrial toxicity
- Biological effects monitoring
- Biotransformation and kinetics
- Additional ecotoxological information
- Toxicological Summary
- Toxicokinetics, metabolism and distribution
- Acute Toxicity
- Irritation / corrosion
- Sensitisation
- Repeated dose toxicity
- Genetic toxicity
- Carcinogenicity
- Toxicity to reproduction
- Specific investigations
- Exposure related observations in humans
- Toxic effects on livestock and pets
- Additional toxicological data
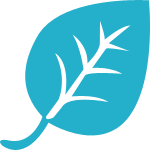
Endpoint summary
Administrative data
Description of key information
Additional information
MITI :
Published Result:
Chemical substance determined to be persistent but not highly bio-accumulative
Judgement: Non-biodegradability
Remarks: None reported
Test Method: Bio-concentration test
Acute Toxicity Test
LC50 (96 hr): > 125 mg/L
LC50 (48 hr): Not Reported
Species: Rice fish (Oryzias latipes)
Flow-through Test
Test Equipment: Improved type for a volatile substance
Test period. 60 days
Species: Carp (Cyprinus carpio)
Lipid Content (%): Not Reported
Lipid Content (%) - start of testing: 4.2
Lipid Content (%) - end of testing: 3.97
Test Concentration - 1st Concentration: 10 microg/L
BCF– 1st Concentration: 200 - 1100
Test Concentration - 2nd Concentration: 1 microg/L
BCF– 2nd Concentration 87 - 310
Partition coefficient(noctanol/ water): not reported
Minimum (log Pow)
Maximum (log Pow)
Average (log Pow)
Brandsma:
The present study did not allow the detection of FTOHs because no validated method was available at the time. Therefore, this study should be regarded as a preliminary range finding investigation, which focused on the non-volatile transformation products in the fish. Exposure to 8:2 and 10:2 FTOHs via food resulted in a series of perfluoroalkyl metabolites (FTCAs and FTUCAs) as shown in Fig. 1. This figure shows the growth-corrected concentrations of FTCAs and FTUCAs in rainbow trout muscle samples over a 30-d dietary exposure to 6.7 lg g1 wet wt 8:2 FTOH and 5.0 lg g1 wet wt 10:2 FTOH, following by a 30-d depuration phase. The predominant metabolites are 10:2 FTCA and 10:2 FTUCA, followed by 8:2 FTCA and 8:2 FTUCA. This is consistent with earlier studies, which observed similar metabolites during exposure of 8:2 FTOH to rats and mice (Hagen et al., 1981; Kudo et al., 2005; Martin et al., 2005; Fasano et al., 2006). The same metabolites were also found in the 8:2 FTOH in vitro metabolism study with trout hepatocytes and the 8:2 FTAc in vivo metabolism study with rainbow trout (Nabb et al., 2007; Butt et al., in press). Butt et al. (in press) found that only low concentrations of 8:2 FTOH were accumulated in rainbow trout tissues following dietary exposure to 8:2FTAc. In addition, Butt et al. (in press) measured an 8:2 FTOH-glucuronide conjugate at relatively high concentrations in the bile and feces of the FTAc exposed trout. Conjugate metabolites (e.g. glucuronide and glutathione) and 7:3 FTCA reported in earlier studies were not measured in this study. The fish extract were only measured for the compounds shown in Table 1. Small quantities of PFOA and PFDA were also detected in rainbow trout exposed to 8:2 and 10:2 FTOH. This indicates that rainbow trout can metabolize FTOH to the more stable PFCAs. The concentrations appear to be very low, just above the detection limit (1 ng g1). Therefore, no calculation could be done concerning uptake and depuration parameters. This biotransformation observed in rainbow trout is consistent with previous animal studies which have shown that small quantities of PFOA and PFNA can be formed after exposure to 8:2 FTOH (24–28). PFNA was not detected in this study. Biotransformation of 8:2 FTAc and 8:2 FTOH to PFOA was also observed in exposed trout and trout hepatocytes, respectively (Nabb et al., 2007; Butt et al., in press) and Butt et al. (2010b) showed FTCAs and FTUCAs formed PFCAs. Butt et al. (in press) found that PFNA was formed in much lower concentrations than PFOA (1%), thus given that PFOA levels were just above the detection limit, the lack of detection of PFNA is not surprising. The concentrations found after 30 d for 10:2 FTCA are significantly higher than those of 8:2 FTCA (Fig. 1). The assimilation and the metabolism of the FTOHs was a relatively fast process detectable after 1 d of exposure. This is consistent with the trout exposure study of Butt et al. (in press) which observed 8:2 FTCA within 1 h after 8:2 FTAc dosing. Nabb et al., 2007 reported halflives after in vitro metabolism of 8:2 FTOH in rat, mouse, trout and human hepatocytes of 9.9, 13, 36 and 103 min, respectively. The fact that 8:2 FTCA and 8:2 FTUCA are almost at steady-state throughout the uptake (Fig. 1) seems consistent with a fast biotransformation of both the 8:2 FTOH, 8:2 FTCA and 8:2 FTUCA. After 10 d the 8:2 FTCA and 8:2 FTUCA decreased to concentrations below the LOD, while this took 20 and 30 d, respectively for the 10:2 FTCA and 10:2 FTUCA; 10:2 FTCA showed the longest half-life (3.7 ± 0.4 d). The others showed half-lives ranging from 1.3 to 3.3 d. Interpretations of the half-lives of 8:2 FTCA and 8:2 FTUCA should be done with care, because the calculations were only possible by linear regression through two growth-corrected concentration points, and no standard error could be calculated. The longer half-lives of the long-chain FTCAs are consistent with the results of Martin et al. (2003a,b). Half-lives reported in the literature for PFOA and PFDA, 5.2 and 14 d, respectively, show that the halflives of the FTCAs are comparable with that of PFOA, and, consequently, lower than that of PFDA. The differences in half-lives between chain-lengths may also explain why the 10:2 FTCA and 10:2 FTUCA were accumulated in greater quantities as compared to the 8:2 FTCA and 8:2 FTUCA.
Information on Registered Substances comes from registration dossiers which have been assigned a registration number. The assignment of a registration number does however not guarantee that the information in the dossier is correct or that the dossier is compliant with Regulation (EC) No 1907/2006 (the REACH Regulation). This information has not been reviewed or verified by the Agency or any other authority. The content is subject to change without prior notice.
Reproduction or further distribution of this information may be subject to copyright protection. Use of the information without obtaining the permission from the owner(s) of the respective information might violate the rights of the owner.
