Registration Dossier
Registration Dossier
Data platform availability banner - registered substances factsheets
Please be aware that this old REACH registration data factsheet is no longer maintained; it remains frozen as of 19th May 2023.
The new ECHA CHEM database has been released by ECHA, and it now contains all REACH registration data. There are more details on the transition of ECHA's published data to ECHA CHEM here.
Diss Factsheets
Use of this information is subject to copyright laws and may require the permission of the owner of the information, as described in the ECHA Legal Notice.
EC number: 237-358-4 | CAS number: 13762-14-6
- Life Cycle description
- Uses advised against
- Endpoint summary
- Appearance / physical state / colour
- Melting point / freezing point
- Boiling point
- Density
- Particle size distribution (Granulometry)
- Vapour pressure
- Partition coefficient
- Water solubility
- Solubility in organic solvents / fat solubility
- Surface tension
- Flash point
- Auto flammability
- Flammability
- Explosiveness
- Oxidising properties
- Oxidation reduction potential
- Stability in organic solvents and identity of relevant degradation products
- Storage stability and reactivity towards container material
- Stability: thermal, sunlight, metals
- pH
- Dissociation constant
- Viscosity
- Additional physico-chemical information
- Additional physico-chemical properties of nanomaterials
- Nanomaterial agglomeration / aggregation
- Nanomaterial crystalline phase
- Nanomaterial crystallite and grain size
- Nanomaterial aspect ratio / shape
- Nanomaterial specific surface area
- Nanomaterial Zeta potential
- Nanomaterial surface chemistry
- Nanomaterial dustiness
- Nanomaterial porosity
- Nanomaterial pour density
- Nanomaterial photocatalytic activity
- Nanomaterial radical formation potential
- Nanomaterial catalytic activity
- Endpoint summary
- Stability
- Biodegradation
- Bioaccumulation
- Transport and distribution
- Environmental data
- Additional information on environmental fate and behaviour
- Ecotoxicological Summary
- Aquatic toxicity
- Endpoint summary
- Short-term toxicity to fish
- Long-term toxicity to fish
- Short-term toxicity to aquatic invertebrates
- Long-term toxicity to aquatic invertebrates
- Toxicity to aquatic algae and cyanobacteria
- Toxicity to aquatic plants other than algae
- Toxicity to microorganisms
- Endocrine disrupter testing in aquatic vertebrates – in vivo
- Toxicity to other aquatic organisms
- Sediment toxicity
- Terrestrial toxicity
- Biological effects monitoring
- Biotransformation and kinetics
- Additional ecotoxological information
- Toxicological Summary
- Toxicokinetics, metabolism and distribution
- Acute Toxicity
- Irritation / corrosion
- Sensitisation
- Repeated dose toxicity
- Genetic toxicity
- Carcinogenicity
- Toxicity to reproduction
- Specific investigations
- Exposure related observations in humans
- Toxic effects on livestock and pets
- Additional toxicological data
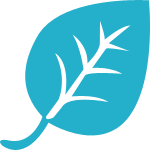
Bioaccumulation: aquatic / sediment
Administrative data
Link to relevant study record(s)
Description of key information
For Cobalt, BCF/BAF valules in the range of 7.4 to 3110 L/kg were reported (mean 878, median 720). All of the 27 BCF/BAF reported for Molybdenum ranged below 100 with the exception of one BAF measured for a mollusc exposed to background Mo water concentrations (BAF of 164). Further data demonstrates that Co and Mo, like other essential elements, show homeostatic control by organisms.
Key value for chemical safety assessment
Additional information
No data for aquatic bioaccumulation are available for cobalt molybdenum oxide. However, there are reliable data available for different structurally analogue substances and for cobalt and molybdenum measured as element in field investigations, respectively.
The environmental fate pathways and ecotoxicity effects assessments for cobalt metal and cobalt compounds as well as for molybdenum metal and molybdenum compounds is based on the observation that adverse effects to aquatic, soil- and sediment-dwelling organisms are a consequence of exposure to the bioavailable cobalt ion and molybdenum ion, released by the parent compound. The result of this assumption is that the ecotoxicology will be similar for all soluble cobalt and molybdenum substances used in the ecotoxicity tests. Therefore, data from soluble cobalt and molybdenum substances are used for the derivation of ecotoxicological and environmental fate endpoints, based on the cobalt ion and molybdenum ion, respectively. With respect to these considerations, data collected on elemental cobalt (e.g. environmental concentrations for Co2+) and molybdenum (e.g. environmental concentrations for Mo2 +) can also be taken into account.
Cobalt
Information taken from Environment Canada (2011):
Considering all aquatic data, 31 acceptable bioaccumulation factors were reported for various species of algae, invertebrates, fish, and zooplankton. These values ranged from 7.4 to 3110 L/kg, with a mean value of 878 L/kg and a median value of 720 L/kg. Five biota-to-sediment accumulation factors (BSAF-sed.) were reported. BSAF-sed values ranged from 0.091 to 0.645, with a mean value of 0.232 and a median value of 0.138 (Environment Canada, 2011).
If marine and freshwater data are pooled, then for aquatic invertebrates, 16 BCF and BAF values were obtained, ranging from 21.8 to 2280 L/kg with an average value of 724 L/kg and a median value of 441 L/kg (wet weight). In comparison, values for fish (n=11) ranged from 7.4 to 3110 L/kg, with an average value of 1010 L/kg and a median value of 849 L/kg. Many studies have noted that homeostatic mechanisms likely exist to regulate cobalt accumulation, due to the fact that it is an essential element (Environment Canada, 2011).
One study, done by Norwood et al. (2006), was unique in its use of a mechanistically-based saturation model for the bioaccumulation of cobalt. The test organism was the freshwater amphipod Hyalella azteca. The wet-weight BCF was calculated according to the equation: BCF = (max)(DW-1)1000K-1, where max is the maximum above-background accumulation of the metal in the organism, measured in nmol/g, DW-1 is the mean dry-to-wet weight ratio for the organism, and K is the half saturation constant (i.e. the metal concentration in the water at which the concentration in the organism is halfway between the maximum and the background accumulations), measured in nmol/L. So, it is seen that this model estimates a BCF based on background-corrected metal accumulation at low aqueous concentrations; thus, unlike with other approaches, background contaminant concentrations will not dictate the BCF values observed. In this case, the wet-weight BCF for Hyalella azteca was found to be 515 L/kg.
In the study carried out by El-Shenawy (2004), metal concentrations were measured in the bivalve Ruditapes decussatus, and in surrounding waters at two different contaminated sites for the calculation of BAFs ranging from 227.1 to 365.7. A lower BAF was observed at the site with a higher ambient cobalt concentration. This inverse relationship between BAF and ambient cobalt concentration provides evidence for the existence of regulation mechanisms in this invertebrate, as previously explained.
Several of the studies used field observations to calculate relevant values. While these data are environmentally realistic, the presence of multiple contaminants, especially other metals, likely influenced the BAF values observed for cobalt. Along these lines, one laboratory experiment by Fraysse et al. (2002) investigated the effect of the presence of cadmium and/or zinc on cobalt accumulation. Two species of freshwater bivalves (Dreissena polymorpha and Corbicula fluminea) were exposed to either cobalt alone, cobalt plus cadmium, cobalt plus zinc, or cobalt plus cadmium and zinc. For D. polymorpha, a BCF of 1100 was determined for whole body wet weight (17 for whole soft body), while for C. fluminea, a BCF of 530 was reported for whole body wet weight (10 for whole soft body). In the end, maximum concentration factors were observed when organisms were exposed to cobalt alone; and, the addition of zinc alone had the greatest inhibitory effect on cobalt uptake (though cadmium and cadmium plus zinc treatments also had an inhibitory affect). Thus, it is important to consider both polymetallic field exposures and controlled laboratory exposures when evaluating cobalt accumulation data.
Biomagnification
Additionally, a study done by Baudin and Fritsch (1989) is referenced. Here, when the carp Cyprinus carpio received cobalt from contaminated food (the mollusc Lymnea stagnalis), the biomagnification factor was reported to have been in the order of 10-2 (though the actual value was not reported). Additionally, it was concluded that water is the dominant pathway for cobalt uptake, and that accumulation from food and water is additive.
Ikemoto et al. (2008) considered the freshwater food web of the Mekong Delta in South Vietnam, examining phytoplankton, snails, five species of crustaceans, and fifteen species of fish. A TMF (trophic magnification factor) for cobalt of 0.95 resulted, but again there was no statistical significance (r2=0.013, p=0.506). Thus the results showed no biomagnification or biodilution of cobalt through the food chain.
There are several lines of evidence to suggest that the bioaccumulation potential of cobalt in natural ecosystems is relatively low. First of all, low BAFs have been reported in eight laboratory (steady state) studies and four field studies; five BSAF-sediment values have been found to be well below 1; and, four (out of four) average BSAF-soil values have been reported to be well below 1. In addition, results from six field investigations plus two laboratory studies indicate the absence of biomagnification of cobalt in natural food webs. Finally, cobalt is an essential micro-nutrient, the uptake of which is expected to be regulated to some extent by many organisms (Environment Canada, 2011).
References:
Environment Canada. Health Canada (2011). Screening Assessment for the Challenge. Cobalt, cobalt chloride, cobalt sulfate.
Molybdenum
The current data set comprises thirty-five values that represent whole body Mo levels in fish (based on wet weight). Values are situated between 0.012 and 14.3 mg/kg wet weight, with a median Mo concentration of 0.22 mg/kg wet weight. Four values below detection limit were not included as the detection limit of <0.5 mg/kg wet weight was greater than the median value. Assuming that levels in these fish were equal to 0.5 mg/kg wet weight, a median value of 0.31 mg/kg wet weight is obtained. The 90th percentile of Mo concentration in whole body samples was 3.00 mg/kg wet weight.
Also available are Mo levels in fish compartments. Regardless of the exposure concentration, Mo levels in livers of fish ranged from <0.019 to 14.9 mg/kg wet weight (n=15), with a median value of 0.45 mg/kg wet weight, and a 90th percentile of 1.71 mg/kg wet weight. Mo levels in muscle samples were markedly lower, i.e., ranging from 0.01 to 7.59 mg/kg wet weight (n=15), with a median value of 0.043 mg/kg wet weight, and a 90th percentile of 0.924 mg/kg wet weight. Median Mo level in the muscle tissue is one order of magnitude lower than the median Mo level in liver samples.
Median levels for other organs like bone (n=4), brain (n=4), intestines (n=4), kidneys (n=4), skin (n=4), spleen (n=5) and stomach (n=4), were 0.185, 0.028, 0.064, 0.1184, 0.071, 0.092 and 0.041 mg/kg wet weight, respectively. For the gill only three data points were identified, ranging from 0.016 to 5.94 mg/kg wet weight.
Whole body Mo levels in crustaceans and other invertebrates ranged from 0.035 to 0.455 mg mo/kg wet weight at background concentration levels in water. At a highly contaminated site, whole body internal concentrations ranged from 2.8 to 32 mg Mo/kg wet weight, although the Mo concentration in the water was not reported.
The data for Mo levels in other organisms (molluscs and phytoplankton) were also generally found below 1 mg Mo/kg wet weight.
Further to the data reviewed and presented here, Eisler (1989) made an exhaustive compilation of environmental concentrations of Mo, including the aquatic compartment. This review concluded also that Mo concentrations in algae, freshwater and marine fish and molluscs are all well below 10 mg/kg dry weight.
Reported whole-body BAFs vary more than 2 orders of magnitude but, as theoretically predicted for essential elements, there is a distinct close relationship between exposure concentration and BAF, i.e., decreasing BAFs with increasing Mo levels in the water column, showing homeostatic control of Mo by these organisms. The homeostatic control of Mo is observed to continue to function up to the milligramme range of exposure.
BCF/BAF could not be derived for all the studies that report internal Mo concentrations in organisms because not all studies report the Mo concentrations in water. From the data where BCF/BAF could be derived, it is noted that BCF/BAF range from 30.1 to 71.6 (average of 49) for fish exposed to background Mo concentrations in water (wet weight basis). The BCF/BAF range from 0.4 to 9.9 (average 1.4) for fish exposed to waters contaminated with Mo (with levels of Mo up to the mg/L range).
BCF/BAF range from 76.2 to 97.6 (average 84) for crustaceans exposed to background Mo concentrations in water (wet weight basis). A BAF of 4.8 was derived for crustaceans exposed to waters contaminated withFor molluscs, the BCF/BAF ranged from 77.3 to 164.3 (average 121) exposed at background Mo concentrations (wet weight basis). No BCF/BAF could be derived for molluscs exposed to Mo contaminated waters.
For phytoplankton a BCF of 20.4 was derived from exposure at background Mo concentrations.
Some information on transfer of Mo through the food chain has been reported by Saiki et al. (1992) and Ikemoto et al. (2008).
In the report by Saiki et al. (1992), highest concentrations of molybdenum were found in detritus and filamentous algae, whereas low concentrations were reported in sediment, invertebrate and fish living in a river containing 10 ug/L Mo in the water. In the study by Ikemoto et al. (2008) the authors measured internal concentrations of Mo in phytoplankton, crustaceans and fish and conclude that there is no biomagnification of Mo across the aquatic foodchain.
These studies indicate that biomagnification of Mo is not significant in the aquatic foodchain.
Information on Registered Substances comes from registration dossiers which have been assigned a registration number. The assignment of a registration number does however not guarantee that the information in the dossier is correct or that the dossier is compliant with Regulation (EC) No 1907/2006 (the REACH Regulation). This information has not been reviewed or verified by the Agency or any other authority. The content is subject to change without prior notice.
Reproduction or further distribution of this information may be subject to copyright protection. Use of the information without obtaining the permission from the owner(s) of the respective information might violate the rights of the owner.
