Registration Dossier
Registration Dossier
Data platform availability banner - registered substances factsheets
Please be aware that this old REACH registration data factsheet is no longer maintained; it remains frozen as of 19th May 2023.
The new ECHA CHEM database has been released by ECHA, and it now contains all REACH registration data. There are more details on the transition of ECHA's published data to ECHA CHEM here.
Diss Factsheets
Use of this information is subject to copyright laws and may require the permission of the owner of the information, as described in the ECHA Legal Notice.
EC number: 807-612-4 | CAS number: 1393645-32-3
- Life Cycle description
- Uses advised against
- Endpoint summary
- Appearance / physical state / colour
- Melting point / freezing point
- Boiling point
- Density
- Particle size distribution (Granulometry)
- Vapour pressure
- Partition coefficient
- Water solubility
- Solubility in organic solvents / fat solubility
- Surface tension
- Flash point
- Auto flammability
- Flammability
- Explosiveness
- Oxidising properties
- Oxidation reduction potential
- Stability in organic solvents and identity of relevant degradation products
- Storage stability and reactivity towards container material
- Stability: thermal, sunlight, metals
- pH
- Dissociation constant
- Viscosity
- Additional physico-chemical information
- Additional physico-chemical properties of nanomaterials
- Nanomaterial agglomeration / aggregation
- Nanomaterial crystalline phase
- Nanomaterial crystallite and grain size
- Nanomaterial aspect ratio / shape
- Nanomaterial specific surface area
- Nanomaterial Zeta potential
- Nanomaterial surface chemistry
- Nanomaterial dustiness
- Nanomaterial porosity
- Nanomaterial pour density
- Nanomaterial photocatalytic activity
- Nanomaterial radical formation potential
- Nanomaterial catalytic activity
- Endpoint summary
- Stability
- Biodegradation
- Bioaccumulation
- Transport and distribution
- Environmental data
- Additional information on environmental fate and behaviour
- Ecotoxicological Summary
- Aquatic toxicity
- Endpoint summary
- Short-term toxicity to fish
- Long-term toxicity to fish
- Short-term toxicity to aquatic invertebrates
- Long-term toxicity to aquatic invertebrates
- Toxicity to aquatic algae and cyanobacteria
- Toxicity to aquatic plants other than algae
- Toxicity to microorganisms
- Endocrine disrupter testing in aquatic vertebrates – in vivo
- Toxicity to other aquatic organisms
- Sediment toxicity
- Terrestrial toxicity
- Biological effects monitoring
- Biotransformation and kinetics
- Additional ecotoxological information
- Toxicological Summary
- Toxicokinetics, metabolism and distribution
- Acute Toxicity
- Irritation / corrosion
- Sensitisation
- Repeated dose toxicity
- Genetic toxicity
- Carcinogenicity
- Toxicity to reproduction
- Specific investigations
- Exposure related observations in humans
- Toxic effects on livestock and pets
- Additional toxicological data
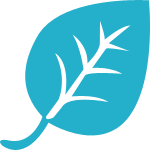
Phototransformation in water
Administrative data
Link to relevant study record(s)
- Endpoint:
- phototransformation in water
- Type of information:
- experimental study
- Adequacy of study:
- key study
- Study period:
- 01-05-2016 to 01-07-2019
- Reliability:
- 2 (reliable with restrictions)
- Rationale for reliability incl. deficiencies:
- comparable to guideline study with acceptable restrictions
- Remarks:
- The study was conducted according to generally accepted scientific principles and sufficiently described
- Study type:
- direct photolysis
- Qualifier:
- according to guideline
- Guideline:
- OECD Guideline 316 (Phototransformation of Chemicals in Water - Direct Photolysis)
- Deviations:
- no
- GLP compliance:
- no
- Remarks:
- In accordance with REACH Regulation (EC) 1907/2006: Annex XI: section 1.1.2 adequate and reliable (with restrictions) study information has been provided (not in accordance with GLP) but which can be considered equivalent to the relevant test method.
- Radiolabelling:
- no
- Analytical method:
- gas chromatography
- high-performance liquid chromatography
- mass spectrometry
- photometric method
- Details on sampling:
- - Sampling intervals for the parent/transformation products: Subsamples were taken at 0, 20, 40, 60, 120, 180, 240, 300, 360 and 480 minutes for analysis by GC-EI/MS
- Sampling method: Sample aliquots of 1 mL were placed in 5 mL disposable glass centrifuge tubes with an equal amount of ethyl acetate, vortexed, allowed to separate, and the top layer of ethyl acetate was transferred with a pipette to a GC vial. The recovery of test item was found to be quantitative by comparative GC-EI/MS analysis with standards in ethyl acetate.
- Sampling methods for the volatile compounds, if any: Not applicable.
- Sampling intervals/times for pH measurements: Not applicable.
- Sampling intervals/times for sterility check: Not applicable.
- Sample storage conditions before analysis: Analysed immediately.
- Other observation, if any (e.g.: precipitation, color change etc.): None reported. - Buffers:
- - pH: 7.0
- Type and final molarity of buffer: Not applicable.
- Composition of buffer: Not applicable.
- Other: Applicant assessment indicates that the test item was not ‘appreciably ionic’ anywhere within the pH 4 to 9 range based on structural assessment. There was also no indications of abiotic degradation (hydrolysis study, 2016)
- Light source:
- Xenon lamp
- Light spectrum: wavelength in nm:
- >= 290 - <= 800
- Details on light source:
- Indoor experimentation:
- Emission wavelength spectrum: 290 nm to 800 nm
- Filters used and their purpose: Yes glass UV filters, to remove light < 290 nm
- Light intensity at sample and area irradiated: 765 W/m2 (Xeon arc lamp setting)
- Relative light intensity based on intensity of sunlight: Not indicated.
- Duration of light/darkness: 80 min in solar simulator
- Other: See attachments for further information.
Outdoor experimentation:
- Location: Plainsboro, NJ (USA)
- Latitude: 40°N
- Longitude: 75°W
- Hours of daylight: 4 hours (10 am to 12 pm) during test item exposure
- Time of year/month: July
- Light intensity: Not indicated.
- Duration of light/darkness: See above.
- Other: See attachments for further information. - Details on test conditions:
- TEST SYSTEM
- Type, material and volume of test apparatus/vessels: quartz test tubes closed with Teflon-lined caps
- Sterilisation method: Not reported.
- Measures to saturate with oxygen: Not reported.
- Details on test procedure for unstable compounds: Not applicable.
- Details of traps for volatile, if any: None.
- If no traps were used, type of test system: Closed.
- Indication of test material adsorbing to the walls of test apparatus: No.
TEST MEDIUM
- Volume used/treatment: ca. 20 mL.
- Kind and purity of water: See any other information on materials and methods and/or any other information on results inc. tables.
- Source of natural water (if applicable) in terms of geographical location, site characteristics and date of collection: See below.
- Preparation of test medium: See below.
- Renewal of test solution: Not applicable.
- Identity and concentration of co-solvent: Not applicable.
- Concentration of solubilising agent: Not applicable.
REPLICATION
- No. of replicates (dark): Duplicate.
- No. of replicates (irradiated): Duplicate. - Reference substance:
- no
- Dark controls:
- yes
- Preliminary study:
- OECD TG 316 (2008) : Tier 1 screening:
The UV-visible absorbance spectrum of the substance was determined: max Lambda = 310 nm (measured absorbance absorbing light at 290 to 380 nm wavelength) and molar absorptivity = 60.0 M-1 cm-1. The substance is therefore is likely to be subject to direct photodegradation.
The substance is stable to abiotic hydrolysis, at neutral pH (ref: hydrolysis (2016)), the UV-visible absorption spectra was measured at pH 7.
A maximum possible direct photolysis rate was calculated to be 12.9 day-1, assuming the quantum yield is equal to one and the average daily solar photon irradiance from a summer day (22nd June) at 50°N latitude. The corresponding half-life was estimated to be 0.054 days. According to OECD TG 316 (2008), since this is << 30d, it was considered relevant to conduct a further experimental phase since photodegradation may have a significant impact on the degradation of the chemical in water. - Parameter:
- max lambda
- Value:
- 310 nm
- Remarks:
- test item
- Quantum yield (for direct photolysis):
- 0.294
- Predicted environmental photolytic half-life:
- See table 1 and table 2.
- Transformation products:
- yes
- Validity criteria fulfilled:
- yes
- Conclusions:
- Under the conditions of the study, the substance is photodegraded under laboratory conditions (quantum yield of 0.294) which was confirmed in outdoor solar experimentation at 40°N latitude. Further modelling and considering the global mean DOC concentration of 5 mg C/L, a latitude of 50°N and a typical mixed water depth of 3 m, it was found that the photodegradation half-life of the test item was 60 times (half-life of 11.2 days) that of clear water near the surface (half-life of 0.19 day) under clear sky expressed as average day of the year. Two photoproducts were detected. Formation of oxidized and more polar products, as well as photo-mineralization, was expected for the test item.
- Executive summary:
The photodegradation (direct and indirect) was investigated using a method equivalent to OECD TG 316 (Phototransformation of Chemicals in Water – Direct Photolysis) and/or utilising additional approaches as detailed in the relevant guidance OECD (1997), and scientific literature. Further environmental fate assessment of the substance was completed within the study using applicable scientific literature, models and/or recommendations (Lin et. al., 2019 and OECD (2007) to extrapolate the half-lives of pure water to simulate realistic conditions, representative of the European continent accounting for natural variations (latitude, season, type and depths in water). Direct photodegradation of the test item in clear water in a solar simulator was determined to be the dominant photodegradation process with a high quantum yield of 0.294. This rapid photodegradation process was corroborated by outdoor experiments conducted under natural sunlight. The quantum yield was used to extrapolate direct photodegradation half-lives in natural waters by considering not only variation of light with latitude and season but also attenuation of light by dissolved organic matter of different depths. Considering the global mean DOC concentration of 5 mg C/L (Thurman, 1985), a latitude of 50°N and a typical mixed water depth of 3 m (as it is used in environmental risk assessment and proposed by Castro Jiménez and Van de Meent, 2011), it was found that the photodegradation half-life of the test item was 60 times (half-life of 11.2 days) that of clear water near the surface (half-life of 0.19 day) under clear sky expressed as average day of the year. Photodegradation products formed were investigated. Two cycloaddition products were detected by GC-MS as main volatile photoproducts of the parent test item. Further photodegradation of the volatile photoproducts was observed. Formation of oxidized and more polar products, as well as photo-mineralization, was expected based on previous work (Lin and Emberger, 2017).
Reference
OECD TG 316 (2008) : Tier 2 testing:
- Applicant assessment indicates that the test item was not ‘appreciably ionic’ anywhere within the pH 4 to 9 range based on structural assessment.
1. Laboratory determination of quantum yield
The quantum yield, i.e. the fraction of amount of reactant consumed or product formed and the amount of photons absorbed, is optional in the OECD TG 316 (2008) but is very useful to determine in order to better estimate the photodegradation rate or corresponding half-life(s).
The quantum yield of test item was determined by comparing the degradation of test item against the actinometer 4-Nitroanisole/pyridine (PNA) in the solar simulator according to equations (16) to (20) within OECD TG 316 (2008).
Furthermore as suggested in OECD (1997) and in the literature (Frank and Klöpffer, (1989)), a photochemical transformation is not dependent of chemical concentration but to the number of photons absorbed by the chemical. Therefore a single concentration of 1 mg/L test item in pure or natural water lower than 10^-4M was utilised. Frank and Klöpffer (1989) utilised a concentration of 10^-6M. The logarithmic plot of direct photodegradation of test item against the actinometer PNA (containing 2.28 mM pyridine). The slope of the line(kc/kPNA) regression line was 0.987 which was utilised in the quantum yield calculation.
Values of Elambda Ilambda from lambda=250 to 400 nm, calculated every 2 nm, were summed for test item and PNA, which resulted in a quantum yield of Phi c=0.294 indicating a high efficiency photochemical process.
2. Verification of the quantum yield by comparing half-lives measured under sunlight on a clear sky day to those derived from quantum yield and sunlight irradiance
To verify theFc obtained in the laboratory, additional photodegradation experiments were conducted by exposing 1 solutions in test tubes to natural sunlight on the roof of a building in documented in the study report (40°N Latitude, USA) in July. This experiment was conducted in duplicate, on a clear sky day. The measured half-lives were then compared to calculated half-liveswhere the irradiance values were obtained from the SMARTS model from 280 to 400 nm at 1 nm increments.
The measured half-lives were 1.29 and 1.30 h and the calculated half-life was 1.27 h, which were in very good agreement. Differences between the measured and calculated half-lives could be due to an increase in the pathlength of light in the test tube from diffraction or reflection or the uncertainty in the irradiance values introduced by using a solar spectrum calculator (Dulin and Mill, (1982). It was concluded that the quantum yield of 0.294 is accurate
3. Calculated photodegradation half-lives in surface waters
Based on the very short half-lives (below 2 hours) observed in laboratory experiment under simulated sunlight and in outdoor experiment under natural sunlight and the high quantum yield of test item, direct photodegradation is expected to be significant for the aquatic environmental fate of the substance. The importance of direct photodegradation will be largely dependent on latitude and season as well as dissolved and particulate organic matter in natural water that can affect the dose of solar irradiance received by dissolved chemicals that undergo direct photodegradation. Therefore, the photodegradation half-lives were calculated considering not only thelatitude and season but also the dissolved organic carbon (DOC) content of water and the mixed layer depth.
Environmental photodegradation half-lives were calculated with the recently published reference irradiance spectra that were calculated using the SMARTS model (Gueymard, 1995) and validated against spectroradiometer data (Apell and McNeill, 2019). To determine the photodegradation half-lives for an average day of the year, the irradiance values were averaged for the four seasons. Additionally, to predict the half-lives in the outdoor experiments, SMARTS was used to model irradiances at 10 am, 11 am and 12 pm with the parameters outlined for the creation of the reference spectra (Apell and McNeill, 2019) and a columnar ozone abundance of 0.1 atmosphere centimetres. The average spectrum of the spectra at the three times was used to predict the half-lives.
Effect of season and latitude- In pure water or for near-surface conditions where light attenuation by DOC is negligible, the photodegradation half-lives of test item, calculated were mostly on the order of hours (see Table 1). The exception was at high latitudes (60°and 70°) in months with low light conditions where photodegradation half-lives up to 8.5 days were calculated. In months where higher latitudes have longer days (e.g., summer in the northern hemisphere), there was little difference between the photodegradation half-lives calculated at these higher latitudes compared to those near the equator. On the yearly average level, the photodegradation half-lives calculated at these higher latitudes were only 2 to 3 times of those near the equator (see Table 1).
Table 1. Predicted near-surface half-lives (days) for direct photodegradation of test item at different northern latitudes in near-surface, clear water conditions.
northern latitudes* |
21-Mar |
21-Jun |
22-Sep |
22-Dec |
Yearly Ave |
|
|
|
|
|
|
30° |
0.23 |
0.17 |
0.23 |
0.45 |
0.24 |
40° |
0.28 |
0.17 |
0.27 |
0.79 |
0.28 |
50° |
0.38 |
0.18 |
0.37 |
1.82 |
0.35 |
60° |
0.57 |
0.20 |
0.54 |
8.4 |
0.46 |
70° |
1.03 |
0.21 |
0.94 |
-- |
0.59 |
*Actual latitudes encompassing the European continent (27°N to 70°N (northern Finland).
The effect of water depth and/or DOC was considered and assessed in table 2.
Table 2. Estimated photodegradation half-lives (in days) of 1 in the mixed layer (for an average day of the year) of natural waters at European latitudes, variable DOC content and at 2 different depths (3m being the typical mixed water depth according to Castro Jiménez and Van de Meent (2011)
[DOC] mg/L |
0 mg C/L |
1.0 |
2.5 |
5 1) |
7.5 2) |
10 |
12.5 3) |
15 4) |
Latitude |
near-surface |
1 m mixed depth |
||||||
30°N |
0.24 |
0.6 |
1.3 |
2.6 |
4.1 |
5.7 |
7.6 |
9.5 |
40°N |
0.28 |
0.7 |
1.5 |
3.1 |
4.8 |
6.8 |
8.9 |
11.2 |
50°N |
0.35 |
0.9 |
1.9 |
3.8 |
6.0 |
8.4 |
11.1 |
14.0 |
60°N |
0.46 |
1.2 |
2.4 |
4.9 |
7.7 |
10.8 |
18.0 |
(18.0) |
65°N |
0.52 |
1.3 |
2.8 |
5.6 |
8.8 |
12.4 |
20.5 |
(20.5) |
70°N |
1.32 |
1.5 |
3.1 |
6.3 |
(9.9) |
(14.0) |
(23.2) |
(23.2) |
[DOC] mg/L |
0 mg C/L |
1.0 |
2.5 |
5 1) |
7.5 2) |
10 |
12.5 3) |
15 4) |
Latitude |
near-surface |
3 m mixed depth 5) |
||||||
30°N |
0.24 |
1.7 |
3.8 |
7.8 |
12.3 |
17.2 |
22.7 |
28.6 |
40°N |
0.28 |
2.0 |
3.8 |
9.2 |
14.5 |
20.3 |
26.7 |
33.7 |
50°N 6) |
0.35 |
2.5 |
3.8 |
11.4 |
18.0 |
25.3 |
33.2 |
41.9 |
60°N |
0.46 |
3.2 |
3.8 |
14.7 |
23.2 |
32.5 |
42.8 |
(54.0) |
65°N |
0.52 |
3.6 |
3.8 |
16.8 |
(26.5) |
(37.2) |
48.9 |
(61.6) |
70°N |
1.32 |
4.1 |
9.3 |
18.9 |
(29.8 |
(41.9) |
(55.1) |
(69.5) |
1) average global DOC (Thurman 1985) and average Northern Finland DOC (Kortelainen, 1993),
2) average European DOC (Sobek et al 2007),
3) average Central/Southern Finland DOC (Kortelainen, 1993),
5) typical freshwater (Castro Jiménez and van de Meent, 2011).
6) proposed average latitude
Italic/Bold: half-life for average DOC in Northern Finland;
Bold: half-life for average DOC in Central/Southern Finland
(In grey): irrelevant for average DOC in Finland
4. Identification of the Photodegradation products
The photoproduct formation of the test item was investigated by GC-MS. The GC-MS chromatograms showed the appearance of two major peaks during the photodegradation experiment and the EI mass spectra of the two photoproducts are identical and shown (in full study report), indicating that they are structurally alike isomers. The structures of the transformation products were elucidated based on chemistry. The formation and degradation of the cycloaddition photoproducts in both pure water and water containing 5.3 mg C/L SRNOM was monitored by integrating the GC-MS peak areas of the total ion chromatograms. As the level of test item in the solutions decreased due to photodegradation, the concentrations of the photoproducts increased and reached their maximums. One difference between the degradation in the two solutions was that the maximum concentrations of the photoproducts in the pure water solution were reached at 60 min, earlier than in the solution containing SRNOM, which was at 120 min. This may be due to the light screening caused by dissolved organic matter, which could slow the photodegradation of test item compared to the pure water solution. Afterword their concentrations began to decline due to direct photodegradation along with additional from degradation of parent test item.
After irradiation for the experimental time period of 8 h, the amounts of total volatile compounds were reduced to about 14% and 17% in pure water and water containing SRNOM respectively, based on GC-MS total ion chromatogram peak areas. It is believed the loss of the volatile photoproducts was due to the formation of non-volatile oxygenated species by photooxidation. The photooxidation process is continuous, leading to photomineralization as demonstrated by LC-HRMS and total organic carbon analyses by Lin and Emberger (2017).
Description of key information
Phototransformation in water : Quantum Yield = 0.294, (pH 7), OECD TG 316 – Tier 1 and Tier 2, non-GLP, 2019
The quantum yield was used to extrapolate direct photodegradation half-lives in natural waters by considering not only variation of light with latitude and season but also attenuation of light by dissolved organic matter of different depths. Considering the global mean DOC concentration of 5 mg C/L (Thurman, 1985), a latitude of 50°N and a typical mixed water depth of 3 m (as it is used in environmental risk assessment and proposed by Castro Jiménez and Van de Meent, 2011), it was found that the photodegradation half-life of the test item was 60 times (half-life of 11.2 days) that of clear water near the surface (half-life of 0.19 day) under clear sky expressed as average day of the year. Photodegradation products formed were investigated. Two cycloaddition products were detected by GC-MS as main volatile photoproducts of the parent test item. Further photodegradation of the volatile photoproducts was observed. Formation of oxidized and more polar products, as well as photo-mineralization, was expected based on previous work (Lin and Emberger, 2017).
Key value for chemical safety assessment
Additional information
Key Study : OECD TG 316 – Tier 1 and Tier 2, non-GLP, 2019 : The photodegradation (direct and indirect) was investigated using a method equivalent to OECD TG 316 (Phototransformation of Chemicals in Water – Direct Photolysis) and/or utilising additional approaches as detailed in the relevant guidance OECD (1997), and scientific literature. Further environmental fate assessment of the substance was completed within the study using applicable scientific literature, models and/or recommendations (Lin et. al., 2019 and OECD (2007) to extrapolate the half-lives of pure water to simulate realistic conditions, representative of the European continent accounting for natural variations (latitude, season, type and depths in water). Direct photodegradation of the test item in clear water in a solar simulator was determined to be the dominant photodegradation process with a high quantum yield of 0.294. This rapid photodegradation process was corroborated by outdoor experiments conducted under natural sunlight. The quantum yield was used to extrapolate direct photodegradation half-lives in natural waters by considering not only variation of light with latitude and season but also attenuation of light by dissolved organic matter of different depths. Considering the global mean DOC concentration of 5 mg C/L (Thurman, 1985), a latitude of 50°N and a typical mixed water depth of 3 m (as it is used in environmental risk assessment and proposed by Castro Jiménez and Van de Meent, 2011), it was found that the photodegradation half-life of the test item was 60 times (half-life of 11.2 days) that of clear water near the surface (half-life of 0.19 day) under clear sky expressed as average day of the year. Photodegradation products formed were investigated. Two cycloaddition products were detected by GC-MS as main volatile photoproducts of the parent test item. Further photodegradation of the volatile photoproducts was observed. Formation of oxidized and more polar products, as well as photo-mineralization, was expected based on previous work (Lin and Emberger, 2017).
Information on Registered Substances comes from registration dossiers which have been assigned a registration number. The assignment of a registration number does however not guarantee that the information in the dossier is correct or that the dossier is compliant with Regulation (EC) No 1907/2006 (the REACH Regulation). This information has not been reviewed or verified by the Agency or any other authority. The content is subject to change without prior notice.
Reproduction or further distribution of this information may be subject to copyright protection. Use of the information without obtaining the permission from the owner(s) of the respective information might violate the rights of the owner.
